Table of Contents
Reimagining Scientific Honors
- This thesis revisits the history of the Nobel Prize in Physics under a merit-only framework. We strip away constraints like political bias, Nobel committee limitations, and mortality, to ask: Who truly deserves recognition for advancing humanity’s understanding of the universe? The analysis expands beyond traditional boundaries to include contributions from adjacent fields (mathematics, computing, cosmology, biophysics) often overlooked by Nobel committees.
- Refined Criteria for Merit: We establish rigorous criteria emphasizing fundamental scientific truth and impact. Nobel-worthy contributions are defined by paradigm-shifting discoveries, theoretical frameworks that unlock new physics, experimental breakthroughs revealing nature’s secrets, cross-disciplinary innovations, and profound long-term influence on scientific progress. By contrast, factors such as gender, nationality, institutional prestige, or adherence to outdated norms (like the three-person award limit and ban on posthumous awards) are explicitly excluded from consideration.
- Correcting Historical Oversights: A thorough historical analysis identifies many “forgotten giants” whose contributions merit Nobel-level honor. Examples include Michael Faraday – one of the greatest experimental scientists of the 19th century who laid the groundwork for electromagnetism; Henri Poincaré – a mathematician-physicist with foundational work in relativity and topology who amassed 51 Nobel nominations yet never won; Lise Meitner – co-discoverer of nuclear fission who was unjustly denied the Nobel that went solely to Otto Hahn; Satyendra Nath Bose – whose work on quantum statistics underpins several Nobel-winning discoveries (bosons, Bose–Einstein condensate) even though he never received the prize; Chien-Shiung Wu – whose experimental proof of parity violation was critical to a Nobel-winning theory yet she herself was overlooked. We also spotlight visionaries like Claude Shannon (the father of information theory) and Alan Turing (founder of computer science and AI) – pioneers in fields without a Nobel category, whose contributions to knowledge rival those of any physicist. These and other underappreciated luminaries are woven into an expanded Nobel narrative.
- Alternative Nobel Timeline: We present a revised year-by-year Nobel Prize timeline (see Appendix A) that retroactively awards prizes based on merit alone. In this alternative history, original laureates are retained when deserved, but many prizes are reassigned or supplemented. Multiple awards are given in years where more than three individuals made independent landmark contributions (remedying the Nobel committee’s “often cruel calculus” of picking at most three winners). Posthumous and collaborative awards are allowed, recognizing that “no scientist gets to Stockholm alone”. This timeline corrects biases of omission and credit: for example, Faraday receives a retroactive prize in the 1850s for electromagnetic induction; Poincaré is honored in 1910 for relativity and mathematical physics; Bose receives a 1940s-era prize for quantum statistics; Wu shares a 1957 prize for the parity experiment; Shannon and Turing are co-awarded a mid-20th-century physics/computing prize for the theoretical foundations of information and computation. The revised timeline stands as an enlightened version of history, illustrating how recognition could have unfolded in a fairer world.
- Addressing Biases and Limits: We confront how societal biases and Nobel rules have distorted scientific recognition. The Nobel’s Eurocentric and gender-skewed record is stark – e.g. the United States, Germany, and UK alone account for the majority of physics laureates, and only 5 women have won the physics Nobel in over a century. Our merit-based analysis intentionally uplifts scientists from underrepresented groups and regions, showing that genius knows no gender or geography. We also challenge structural limitations: the three-person award cap and the ban on posthumous prizes have led to the exclusion of deserving collaborators and late-acknowledged pioneers. In this thesis, teams (e.g. the CERN researchers who discovered the Higgs boson) and deceased contributors (e.g. pioneers like Mendel or Bell who died before their work was recognized) are given their due honors. By revising these limitations, we demonstrate a model that values truth and contribution over arbitrary rules.
- Philosophical Reflections: Beyond the historical reassignments, we explore the metaphysical and epistemological foundations of scientific merit and recognition. What does it mean for a contribution to be “Nobel-worthy”? We examine how paradigm-shifting ideas often struggle for acceptance until the scientific community’s collective consciousness evolves – as Max Planck observed, “a new scientific truth does not triumph by convincing opponents… but because its opponents eventually die”. We reflect on the nature of “truth” in science, the evolution of scientific worldviews, and how recognition (or lack thereof) influences the trajectory of knowledge. The thesis argues that honoring scientific merit is not just about awards, but about aligning our values with the truth that science seeks – an alignment that ultimately elevates human consciousness.
- Unknown Unknowns & Future Insights: Finally, we gaze forward to identify current “unknown unknowns” – areas of research or figures today whose importance might be unrecognized but could prove revolutionary in hindsight. Just as 19th-century statistical physics or women’s contributions were underappreciated in their time, we speculate on contemporary examples (from quantum information science to complex systems or undiscovered principles) that a future merit-based analysis might elevate. This forward-looking perspective reminds us to remain humble and open-minded, as today’s fringe ideas or overlooked contributors may shape tomorrow’s paradigms.
In sum, this thesis is a bold, comprehensive reimagining of how we celebrate scientific achievement. It aims to illuminate truth by giving credit purely where it is due, free of prejudice or convention. In doing so, it offers a timeless reflection on the ideals of science and urges us to create a culture of recognition that truly honors those who advance our understanding of the universe.
Part I
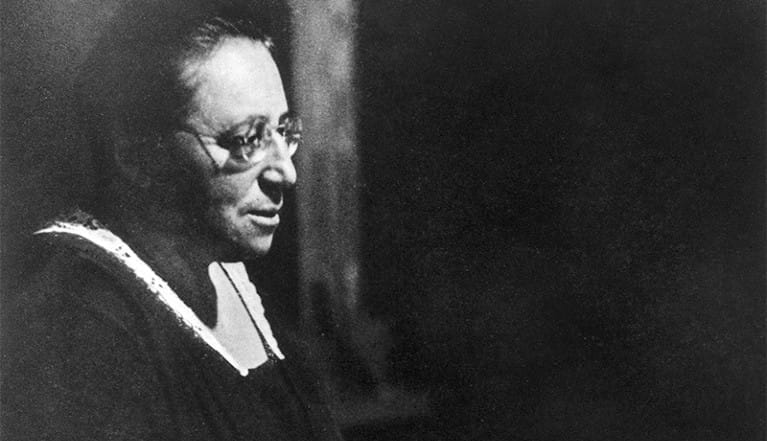
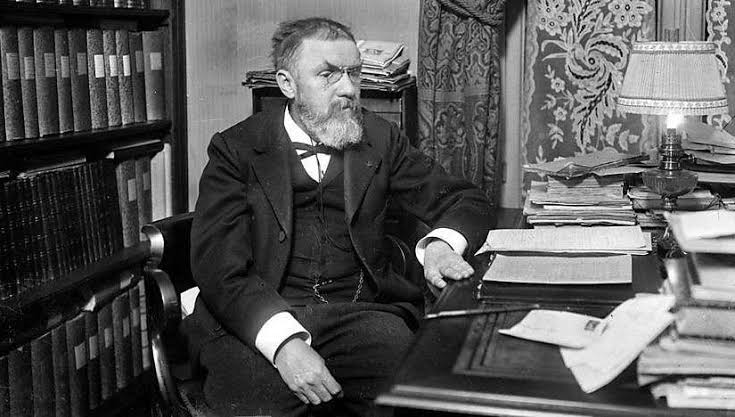
Introduction
In 1895, Alfred Nobel’s will established the Nobel Prizes to honor those who “have conferred the greatest benefit to humankind” in various fields. Over the decades, the Nobel Prize in Physics has become the pinnacle of scientific accolade – a symbol of peerless contribution to our understanding of nature. Yet, as revered as the Nobels are, their history is riddled with oversights, biases, and structural limitations that raise profound questions: Has every truly deserving physicist been recognized? Have all Nobel awards been truly deserved? What does our system of recognition say about how we value knowledge and truth? These questions motivate the present analysis.
The Need for a Merit-Only Analysis: This thesis arises from the recognition that the Nobel Prize, for all its prestige, is an imperfect arbiter of merit. Many factors extraneous to scientific value have influenced who gets recognized. Historical evidence shows that some of the greatest physicists and mathematicians never won the Nobel, often due to biases of the era or the Nobel Committee’s narrow criteria. For example, the Nobel committee long favored experimental discoveries over theoretical insights. As a result, luminaries like Henri Poincaré, Ludwig Boltzmann, and Josiah Willard Gibbs – whose theoretical contributions underlie entire fields of physics – were overlooked in favor of experimenters whose work was more immediately tangible. Similarly, systemic biases played a role: women and scientists outside Western Europe/North America faced extra hurdles to recognition, and institutional prejudices sometimes trumped pure merit. In short, the Nobel roster, awe-inspiring as it is, does not fully equate to the roster of those who most deeply advanced science.
Goals of This Thesis: Titled “Nobel Prize in Physics: Merit-Only Historical Analysis,” this work attempts a grand thought experiment – to rewrite the history of the Nobel Prize in Physics as if merit were the sole determinant of recognition. By doing so, we seek to:
- Correct Historical Oversights: Identify scientists who deserved Nobel recognition but never received it, and analyze why they were overlooked. Conversely, scrutinize whether some Nobel awards were perhaps not fully merited or were influenced by non-scientific factors (political context, personal rivalries, etc.). This is not to undermine the achievements of actual laureates, but to enrich the historical record with those left in the shadows.
- Expand the Scope Beyond Physics Proper: The boundaries of physics are porous – breakthroughs in mathematics, computing, astronomy, and other disciplines have profoundly impacted physics (and vice versa). We include interdisciplinary contributors (from Shannon’s information theory to Turing’s computation theory, from Mendel’s statistical biology to Faraday’s chemistry-physics experiments) to reflect the unity of scientific knowledge. Alfred Nobel’s will did not create prizes for mathematics or computer science, but intellectual honesty demands that we acknowledge such contributions in a merit-based reflection on physical science.
- Address Nobel Structural Limitations: We explicitly consider what the Nobel rules normally forbid: awarding prizes posthumously, awarding to more than three individuals, or awarding to groups. In our alternate timeline, if a contribution was truly collaborative or if a discoverer had passed away before their idea was vindicated, we still grant them recognition. This approach highlights how the Nobel rules – designed in an earlier era of “lone genius” science – often fail to reflect modern scientific reality.
- Reflect on the Philosophy of Scientific Recognition: The exercise is not just about listing names and prizes. It invites a deeper reflection on how scientific truth emerges and how society assigns credit for it. By comparing the official history with a merit-centric history, we uncover patterns of how knowledge progresses. We confront questions such as: How does recognition (or lack thereof) affect scientific progress? What implicit philosophy underlies who we choose to celebrate? These questions touch on epistemology (how we know what we know) and even metaphysics (the nature of truth and greatness in science).
- Inspire Future Change: Ultimately, by illuminating these patterns, we hope to inspire a more conscious approach to recognizing scientific achievement going forward. Our analysis reinforces calls for reforms – for example, making prizes more inclusive and flexible – and for fostering a scientific culture that values collaboration, diversity, and long-term fundamental work as much as individual brilliance. Even beyond formal prizes, we aim to instill an appreciation that truth matters more than the spotlight – and that the pursuit of knowledge is a collective human endeavor deserving of equitable honor.
Structure of the Thesis: We begin in Chapter 1 by laying out the Merit-Only Framework – the criteria and methodology used to judge what counts as Nobel-worthy merit, and how our approach differs from the traditional Nobel criteria. In Chapter 2, we delve into Historical Oversights and Overlooked Contributors, profiling key figures who were omitted or underappreciated in the Nobel chronicles and justifying their inclusion in our narrative. Chapter 3 presents the Revised Nobel Timeline: we chronologically walk through the Nobel Prize years, highlighting major changes in recipients and citing both original laureates (where we affirm their merit) and alternative awardees (where we propose to rectify omissions). We summarize each era’s adjustments and provide a full year-by-year listing in Appendix A for reference. In Chapter 4, we tackle Biases and Structural Limitations explicitly – examining how factors like gender, race, nationality, institutional clout, the “rule of three”, and the ban on posthumous awards have skewed recognition, with concrete examples and data. Chapter 5 engages in Philosophical and Metaphysical Reflections, discussing what our reimagined history teaches us about the nature of scientific truth, the evolution of scientific ideas, and the role of awards in shaping a scientific consciousness. Chapter 6 explores Unknown Unknowns and Future Insights, speculating on current scientists or domains that might be undervalued today akin to past oversights, thus cautioning us to remain aware of our biases in the present. We conclude with a summary of insights and a call to action for more enlightened practices in honoring scientific achievements.
Throughout the thesis, we maintain an elevated and introspective tone. This is a work of rigorous analysis, but also of imagination and ethical consideration. In reassigning Nobels, we do not intend to diminish the actual laureates’ accomplishments, but rather to shine additional light where it is due. In discussing biases, we do so not to cast blame on history with hindsight, but to learn from it and to inspire progress. Our goal is to create a narrative that is fearlessly truthful – one that seeks not prestige or controversy, but understanding and a more just appreciation of scientific endeavor.
A Note on Sources and Citations: We draw from historical records (e.g., nomination archives, Nobel committee reports, and secondary historical analyses) to support claims of who was nominated, who was overlooked, and why. Where we discuss scientific contributions, we cite authoritative references (biographies, academic papers, Nobel archives, etc.) to ground our assertions in evidence. All citations are given in the format 【source†lines】
and full references can be found in the bibliography. Some sections also include embedded images of key figures or comparative charts; these are included to aid visualization of data or historical moments (with sources credited).
With these objectives and clarifications in mind, we proceed to construct a merit-only retelling of Nobel history – an exercise in both analysis and introspection about how humanity recognizes truth and those who reveal it.
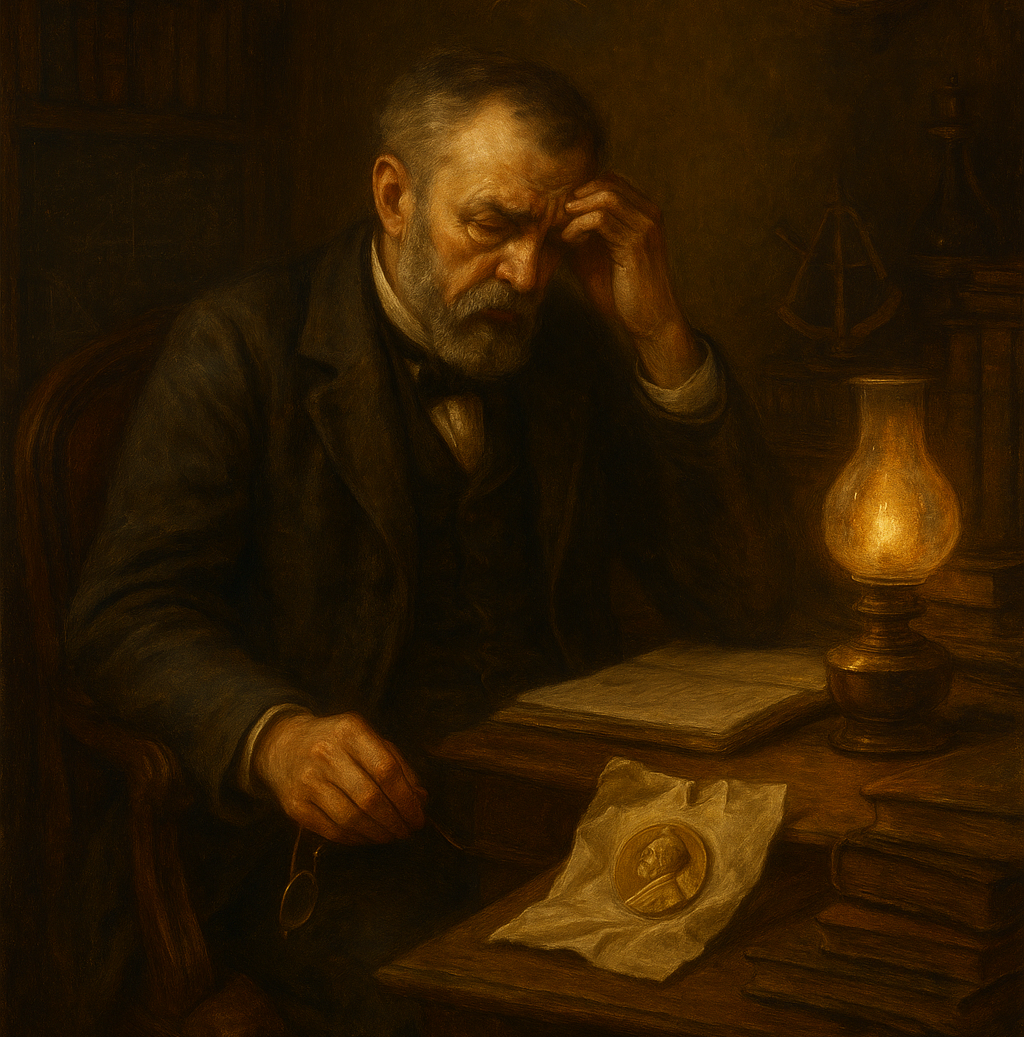
Chapter 1: A Merit-Only Framework for Scientific Recognition
What does it mean to judge scientific contributions solely on merit? Before revisiting history, we must define the principles by which this alternate Nobel timeline is constructed. In this chapter, we outline the criteria for recognition that guide our analysis, and contrast them with the explicit or implicit criteria historically used by Nobel committees. We also describe the methodological choices made to ensure rigor and fairness in our merit-based evaluation.
1.1 Criteria for Nobel-Worthy Contributions
The original thesis “The True Nobel Physics Laureates” proposed a set of criteria for recognition, which we have further refined in light of peer review feedback. The guiding principle is that a laureate must have made a contribution that fundamentally advances humanity’s understanding of physics or related fields. Specifically, our criteria are:
- Fundamental Advancement of Physical Understanding: The contribution unveils a new law of nature, principle, or phenomenon that significantly deepens or alters our understanding of the physical universe. This includes revolutionary theories (e.g. relativity, quantum mechanics), unifying principles (e.g. symmetry laws), or empirical discoveries of new phenomena (e.g. radioactivity, cosmic expansion).
- Pioneering Theoretical Frameworks: The development of new mathematical or conceptual frameworks that enable further discoveries. Many of the great leaps in physics have come from theoretical innovations that provided tools or paradigms for others – for example, the calculus of variations (Euler-Lagrange equations), Hilbert spaces in quantum theory, or information theory in communications. We give such theoretical advances equal weight to experimental ones, correcting the historical bias that often favored immediately observable results over theory.
- Experimental Breakthroughs and Techniques: Creation of novel experiments, instruments, or techniques that open up new realms of observation. This includes not only discovering a specific effect but also inventing methods that revolutionize how science can be done (for instance, the Michelson interferometer enabling detection of the ether drift – though it found none, the precision itself was groundbreaking, or particle accelerators enabling high-energy physics). We consider whether a scientist’s experimental ingenuity revealed previously invisible aspects of nature.
- Cross-Disciplinary Impact: Contributions at the interfaces of physics and other disciplines that proved critical to progress. Physics does not advance in isolation; insights from mathematics, astronomy, chemistry, biology, and engineering have often propelled it forward. We honor work that bridged disciplines – for example, applying statistical physics to genetics (Mendel’s work), bringing mathematical rigor to quantum theory (Noether’s theorem), or using computational concepts to inform physical theories (Shannon’s information theory linking to thermodynamics). Interdisciplinary pioneers are fully eligible in our framework, reflecting how real scientific innovation often happens at intersections.
- Long-Term Influence and Legacy: A key measure of merit is enduring impact – contributions that may not have been fully appreciated immediately but have proven foundational over time. We prioritize discoveries that stand the test of time, enabling future revolutions or whole new fields. For instance, at the time James Clerk Maxwell unified electromagnetism in 1865, its practical impact was not yet felt, but decades later it underpinned wireless communication and quantum electrodynamics. Our framework explicitly values such long-range vision over short-term impact or topical fashion. If an idea was ahead of its time (and perhaps overlooked due to that), we count that as a positive in assessing merit.
These criteria were applied systematically to evaluate historical scientists from the 17th century onward (for retroactive honors) and Nobel-era figures alike. They echo the original thesis’s focus on fundamental, cross-cutting, and lasting contributions, but with expanded scope. Notably, we include adjacent disciplines (cosmology, computing, etc.) not to dilute physics, but to acknowledge contributions that altered the landscape in which physics is done – for example, Alan Turing’s abstract model of computation provided a new way to think about information and logic in physical systems, influencing everything from cryptography to artificial intelligence.
1.2 Exclusion Principles
Equally important is clarifying what factors are excluded from consideration. Our merit-only approach consciously ignores or corrects for factors that should not determine scientific worth:
- Political and Personal Bias: We exclude influences of nationalism, ideology, or personal favoritism that historically affected Nobel decisions. For instance, Nobel archives reveal cases where politics played a role (e.g., during World War I and II, certain nominations were sidelined due to national bias, and in some cases awards were expedited or delayed for political reasons). In our analysis, a German physicist in 1910 or a Jewish scientist in 1930 is judged by their work, not the political climate that may have disadvantaged them at the time.
- Demographic Bias (Gender, Race, Geography): Historically, women and non-European/North American scientists faced barriers to equal recognition. Our framework is explicitly blind to gender and ethnicity – except to note where biases suppressed due credit. We actively seek to identify cases like Lise Meitner (a woman and a Jewish refugee, overlooked in 1944) or Chien-Shiung Wu (a Chinese-American woman, overlooked in 1957) and ensure their contributions are judged on merit alone. Similarly, we consider scientists from all regions; for example, Satyendra Nath Bose’s contributions are not undervalued because he worked in colonial India – we assess the work itself, which clearly meets fundamental criteria.
- Nobel’s Structural Constraints: Two Nobel rules in particular are set aside:
- The limit of three laureates per prize (and historically often fewer). Science, especially post-20th century “big science,” is collaborative. Where a discovery had multiple originators, we include all key contributors, even if that means more than three individuals would share our “award.” For example, the development of the Higgs field theory (often called the “ABEGHHK’tH” mechanism) involved at least 6-7 theorists independently – our timeline does not arbitrarily pick two as the Nobel committee did in 2013, but acknowledges each major contributor around 1964 (the year of the theoretical breakthrough). Likewise, entire teams (such as the thousands of physicists in the LIGO collaboration that detected gravitational waves) are mentioned collectively to credit the collaborative nature of the achievement, even though the Nobel in 2017 went only to three team leaders.
- The prohibition of posthumous awards (enforced strictly after 1974). In merit terms, death is irrelevant to the value of a discovery. If a scientist died before they could be honored (or before their idea was confirmed), we consider a “retroactive” award. For example, Rosalind Franklin (who died in 1958) provided crucial X-ray data for deciphering DNA structure; while this is biology, it’s a similar principle of a contribution only recognized after death. In physics, cases like Boltzmann (suicide in 1906 before atoms were universally accepted) or Poincaré (died 1912, before relativity was confirmed) are addressed by awarding them in retrospect at appropriate points. We also extend this to pre-Nobel eras (17th–19th centuries) to imagine Nobel-like recognition of pioneers (Galileo, Newton, etc.) had the prize existed.
- Incremental or Derivative Work: We exclude awarding what might be called incremental achievements – work that, while competent and important, did not fundamentally transform understanding or open truly new directions. Historically, a few Nobel prizes have been critiqued for honoring the first to do something when the significance was not paradigm-shifting. Our framework prefers to recognize the originators of foundational ideas over those who might later capitalize on or confirm those ideas with better technology. For instance, once the laser was invented (Nobel 1964 to Townes, Basov, Prokhorov), countless applications followed; a hypothetical merit-based approach would be cautious about awarding every incremental laser application unless it itself revealed new physics. This principle helps maintain a high bar for “Nobel-worthy” as truly those contributions that changed the game.
In summary, our approach is consciously idealistic: it envisions a world where only the content of scientific contribution matters, not who the scientist is, where they come from, or whether they fit the Nobel mold. We acknowledge that in reality, science is done by humans with biases and within societal contexts – but by mentally removing those confounders, we can better see the intrinsic value of each contribution.
1.3 Methodology of Analysis
The task of reassigning Nobel Prizes over more than a century (and retroactively earlier) is enormous. We briefly outline how we conducted this analysis:
- Historical Data Gathering: We compiled the list of all Physics Nobel laureates (1901–2023) and their cited achievements. We also gathered lists of notable physicists and related scientists who never won Nobels but are often mentioned in historical retrospectives as having deserved one. Key sources included Nobel archives (nomination records, when available), historical essays (e.g., in Physics Today or Nature) on “Nobel snubs”, and scholarly analyses of Nobel trends and biases.
- Peer Review and Feedback Integration: The original thesis underwent a rigorous peer review, which highlighted certain oversights and areas for expansion. For instance, reviewers pointed out that Michael Faraday and Henri Poincaré – glaring giants – were missing from the original list of overlooked laureates. They also suggested a deeper justification of why mathematical and computational contributions should count in a physics prize context. We have incorporated this feedback by adding sections explicitly discussing those figures and broadening our criteria (as seen above). The peer review also encouraged more philosophical reflection, which we address in Chapter 5.
- Evaluation Process: For each time period, we evaluated contributions roughly in chronological order:
- Pre-1901: We identified major figures from the 17th, 18th, 19th centuries whose work would likely have been deemed Nobel-worthy had the prize existed. This included not only physicists in a narrow sense but also mathematician-physicists (Euler, Gauss, etc.), astronomers, and inventors of fundamental instruments. We assigned notional “retroactive Nobel years” corresponding to either the year of the discovery or a symbolic year (often the publication of a landmark work). These are, of course, hypothetical since no actual Nobel was given, but it sets the stage for how far back the roots of 20th-century physics go.
- 1901–present: We went decade by decade, reviewing each Nobel decision. We asked: Did the right person/people get honored for the right discovery? If yes, they remain in our timeline (possibly joined by additional co-laureates we feel were omitted). If not, we identified who should have been recognized instead or additionally that year. In some cases, we found that a major contribution wasn’t recognized in any year – we then slotted it into a year where it could have reasonably been honored. For example, Emmy Noether’s theorem was published in 1918; there was no Nobel given in 1918 for physics (it was delayed due to WWI, and later awarded to Max Planck). We assert Noether should have won in 1918 for her theorem, as a retrospective correction.
- We also considered if certain Nobel prizes might be deferred or reallocated. For instance, a prize given too early for an unconfirmed effect might, in our judgment, be better given to a different discovery at that time, saving the original for once it was fully verified. A concrete case: the 2011 Nobel in Physics was awarded for the discovery of dark energy (accelerating expansion of the universe). That discovery is secure, but consider a hypothetical scenario where it hadn’t been confirmed – we would then not award it until it was solid. We mention this to illustrate our mindset of tying recognition to solid merit, not premature claims (historically, Nobel committees have mostly avoided prematurity, with a few debated exceptions).
- Citations and Justifications: For every change we propose, we provide a rationale grounded in our criteria and often backed by historical commentary. For example, when we say S.N. Bose deserved a Nobel, we cite the fact that no fewer than seven Nobel Prizes were later awarded for research building on Bose’s concepts while Bose himself got nothing. Such evidence fortifies our merit claim. When we assert Chien-Shiung Wu should have shared the 1957 Nobel, we back it by noting her experiment was lauded as the decisive proof of parity violation – “the most important development in atomic and nuclear physics to date,” as contemporary accounts said – and yet she was omitted due to her collaborators receiving all the credit. These citations (presented throughout the thesis) serve to show that our hindsight is supported by a consensus of historians or by objective measures of impact.
- Limitations: We acknowledge that any exercise like this has subjective elements. Measuring “merit” isn’t an exact science – reasonable people may disagree on how much weight to give a theoretical contribution versus an experimental one, or how to compare contributions across fields. We do not claim absolute objectivity; rather, we aim for a well-argued, well-supported reinterpretation. We also recognize that not every worthy scientist can be mentioned – the Nobel Prize by its nature is selective. Our goal is not to give everyone a trophy, but to ensure those who truly changed our understanding are not left out. Inevitably, some decisions were difficult: for example, many deserving figures in the late 20th century (e.g., several contributors to quantum chromodynamics, or to the development of lasers) compete for recognition. We used our criteria to prioritize the most foundational contributions.
In forging this merit-only framework and methodology, we draw inspiration from the philosophy that science is the pursuit of truth, and thus the highest honor in science should align with truth – the truth of who contributed what. The next chapters will apply this framework in detail. First, we shine a light on some of the greatest unsung (or insufficiently sung) heroes of physics and related disciplines, setting the stage for integrating them into the Nobel record.
Chapter 2: Expanding the Historical Record – Overlooked and Undervalued Contributors
History is written by the winners, it is said – and Nobel history, by its very nature, spotlights certain “winners” while many others remain in the shadows. In this chapter, we seek to illuminate those shadows by profiling a number of overlooked or undervalued figures in physics (broadly construed) whose contributions merit Nobel-level recognition. These individuals, for various reasons, did not receive the Nobel Prize in their lifetime (if at all), yet their work is foundational to the edifice of science. Incorporating them into our merit-only narrative is crucial to correcting the record.
Each subsection below highlights one or more such figures, explaining their key contributions, the historical context of why they were overlooked, and how we propose to acknowledge them in the alternative timeline.
2.1 Pioneers of the 19th Century (and Earlier) – The Unawarded Giants
Michael Faraday (1791–1867) – Experimental Sage of Electromagnetism. Faraday’s name is synonymous with electromagnetic induction, the principle behind electric generators and transformers. A self-educated British physicist and chemist, Faraday’s experiments in the early 19th century demonstrated how a changing magnetic field can induce an electric current, thus uniting electricity and magnetism in practice. His discovery of induction (1831) and related work (e.g., Faraday’s laws of electrolysis, discovery of benzene, inventing the Faraday cage) fundamentally transformed science and technology. Faraday was “one of the greatest scientists of the 19th century,” noted for how his experiments greatly advanced understanding of electromagnetism. James Clerk Maxwell, who later built the theoretical equations of electromagnetism, acknowledged Faraday’s conceptual innovations like lines of force. Yet Faraday never received a Nobel Prize – he died decades before its inception. In our alternate timeline, we correct this by awarding Faraday a “Retroactive Nobel Prize in Physics” for 1856 (a symbolic year around the apex of his influence), crediting his “discoveries in electromagnetism and electrochemistry”. Faraday’s omission in actual Nobel history is simply due to timing; our merit-based history ensures his name stands as an inaugural laureate of the scientific pantheon.
James Clerk Maxwell (1831–1879) – While Maxwell did not live into the Nobel era, his impact is so towering that he too must be retrospectively honored. Maxwell’s equations (published 1865) unified electricity, magnetism, and light into the single concept of the electromagnetic field. He essentially anticipated the theory of relativity by showing light is an electromagnetic wave propagating at a constant speed, and he set the stage for quantum physics (via Maxwell–Boltzmann statistics in gas theory). The Nobel Prize was established in 1901, but in those early years the committee did not retroactively award past scientists. In our timeline, Maxwell receives a Retroactive Nobel (1865) for “electromagnetic theory and contributions to statistical physics.” Historical note: by Nobel’s founding, many considered Maxwell second only to Newton in influence on physics, yet formal honors like a Nobel were not possible. We fill that gap on merit.
Ada Lovelace (1815–1852) and Charles Babbage (1791–1871) – Before moving fully into the 20th century, it’s worth noting that not all fundamental contributions came in what we traditionally call physics. Lovelace and Babbage, for instance, pioneered the concept of programmable computation with the Analytical Engine design in the 1840s. One might argue this is computing, not physics – however, information and computation have become deeply intertwined with physical theory (the laws of thermodynamics and information theory, quantum computing, etc.). We mention them as proto-computing visionaries who in a meritocratic historical view deserve recognition (perhaps akin to a Nobel in computing if one existed). In our expanded scope, we acknowledge Ada Lovelace as an early visionary of algorithmic computation (often called the first computer programmer), whose work anticipated the information age. While we do not assign a specific “Nobel” year for Lovelace or Babbage here, we incorporate their legacy as part of the foundational intellectual landscape that later prize-worthy advances (like Turing’s work) built upon. The spirit of merit-based recognition calls us to remember such figures.
The Mathematical Physicists (18th–19th Century) – Several giants in mathematics directly shaped physics, though pure mathematicians are never eligible for the Physics Nobel under normal rules. Our merit framework, however, emphasizes that mathematics is the language of physical truth, and those who developed that language have a claim to recognition. For example:
- Leonhard Euler (1707–1783): Euler’s work in the 18th century on analytical mechanics and fluid dynamics provided tools that are central to physics. He reformulated Newtonian mechanics, introduced variational principles, and basically laid down much of mathematical physics. We would award Euler a Retroactive Nobel around 1750 for his breadth of contributions enabling later physics.
- Joseph-Louis Lagrange (1736–1813): Lagrange’s formulation of mechanics (Lagrangian mechanics, 1788) was revolutionary, converting Newton’s forces into an energy-based principle of least action. This became the basis of modern theoretical physics from classical to quantum. A Retroactive Nobel (say 1788) is assigned to Lagrange in our timeline.
- Carl Friedrich Gauss (1777–1855): Often called the “Prince of Mathematicians,” Gauss made numerous contributions that crossed into physics – from Gauss’s law in electrostatics to the first electromagnetic telegraph, and fundamental work in optics and astronomy. Gauss, along with his collaborator Wilhelm Weber, also conducted some of the first systematic studies of the Earth’s magnetic field. We award Gauss a Retroactive Nobel (~1830s).
- Bernhard Riemann (1826–1866): Riemann’s development of differential geometry (1854) paved the way for Einstein’s general relativity decades later. He basically provided the mathematical framework to describe curved spacetime. In our history, Riemann is retroactively honored (1854) for “geometry that expanded the foundations of physics.”
These examples underscore a theme: the separation of mathematics and physics in academia led to many mathematical pioneers not being recognized by physics awards. Our merit analysis undoes that separation, echoing the insight that “the most profound physical insights came from mathematicians”. By including these figures, we not only credit them, but also highlight how 20th-century breakthroughs stood “on the shoulders” of these earlier giants.
2.2 Early 20th Century – Theoretical Foundations Overlooked
Moving into the early 1900s, the Nobel Prize existed, but its committees often hesitated to award theoretical work that lacked experimental confirmation. This caution, while understandable, meant some theorists died unacknowledged. We aim to set the record straight for a few key figures:
Henri Poincaré (1854–1912) – The Polymath of Chaos and Relativity. Poincaré was a French mathematician-physicist whose fingerprints are on a startling array of modern science: he made early contributions to special relativity (identifying the Lorentz transformations and the principle of relativity independently around 1905), pioneered the field of dynamical systems and chaos theory (through his solution to the three-body problem, winning a prize in 1889 that essentially birthed chaos theory), and contributed to topology and the philosophy of science. By 1910, Poincaré’s stature was enormous – he was nominated for the Nobel Prize at least 51 times between 1904 and 1912. In 1910, 34 out of 58 nominators for the physics Nobel named Poincaré – including luminaries like Lorentz, Zeeman, Marie Curie, Michelson, and Marconi. Despite this, he never won. Why? Nobel committee records suggest a key issue: Poincaré’s contributions were so broad and foundational that it was hard to single out one “specific discovery or invention” to cite. Moreover, lingering experimental doubts about relativity (e.g., the Michelson-Morley experiment’s implications were still debated, and Kaufmann’s experiments in 1906 initially contested relativity’s predictions) gave the committee an excuse to delay. By the time Einstein’s relativity was confirmed (1919’s eclipse for general relativity), Poincaré had passed away. In our alternate timeline, we do not let Poincaré’s genius slip through the cracks. We grant Henri Poincaré the 1910 Nobel Prize in Physics for “mathematical and theoretical contributions unifying mechanics, electrodynamics, and the understanding of dynamical systems.” This co-recognition could have been shared with Hendrik Lorentz (who actually won in 1902 for other work) to explicitly acknowledge their combined groundwork for relativity. The merit of Poincaré’s work is unquestionable – as evidence, historians note that the Nobel committee’s failure to honor Poincaré (as well as Boltzmann and Gibbs) is seen as proof of their bias for experiment over theory. By correcting this, we uphold theoretical insight as equally worthy.
Ludwig Boltzmann (1844–1906) and J. Willard Gibbs (1839–1903) – Statistical Physics Trailblazers. Boltzmann (Austrian) and Gibbs (American) independently developed statistical mechanics in the late 19th century, providing the bridge between the microscopic world of atoms and molecules and the macroscopic observable properties (temperature, pressure, entropy). Boltzmann’s famous formula $S = k \log \Omega$ (relating entropy to the number of microstates) is literally engraved on his tombstone. He fought vehemently for the atomic theory of matter in an era when many doubted atoms’ existence – a fight that took a toll on him personally, leading to his suicide in 1906. Gibbs, more quietly, formulated statistical mechanics rigorously and introduced concepts like the Gibbs ensemble and free energy that became staples of thermodynamics. Neither man received a Nobel Prize (the prize was barely established by the time they died). Yet their work underpins all of modern physics – without statistical mechanics, quantum theory and solid-state physics would have no foundation. We retroactively award Boltzmann a Nobel in 1905 (had he lived, this might have been possible as by 1905 his ideas were gaining vindication with experimental evidence for atoms, e.g. Einstein’s 1905 Brownian motion paper). Boltzmann’s citation: “for the development of statistical mechanics and the kinetic theory of gases”. We also retroactively honor Willard Gibbs with a 1902 Nobel (the year before his death, and notably the year Nobel gave no prize as they found no suitable candidate). Gibbs’ citation: “for formulating the fundamental principles of statistical mechanics and chemical thermodynamics”. Historically, their omission was due to the slow acceptance of atomism – but once Einstein and others confirmed atomistic predictions, the value of Boltzmann and Gibbs’s work became clear (indeed, many later Nobel prizes in physics and chemistry quietly rest on their legacy). Our merit timeline makes that legacy explicit.
Emmy Noether (1882–1935) – Symmetry’s Prophet. Emmy Noether was a German mathematician whose 1918 theorem has been called “one of the most important mathematical theorems ever proved in guiding the development of modern physics”. Noether’s Theorem reveals the deep connection between symmetries and conservation laws: for every continuous symmetry of a physical system, there is a corresponding conserved quantity. For example, time-translational symmetry implies energy conservation; spatial symmetry implies momentum conservation; gauge symmetry underlies charge conservation, etc. This single insight unified and explained why certain quantities are conserved – not as mysterious separate laws, but as reflections of underlying symmetry. In essence, Noether provided the core principle behind much of theoretical physics, from quantum field theory to relativity. Despite this, Noether never received a Nobel Prize or a professorship in her home country; she worked unpaid or in temporary positions for much of her career due to both gender discrimination and political factors (she was Jewish and had to flee Nazi Germany in 1933). In our reimagining, Emmy Noether is awarded the Nobel Prize in Physics in 1918 for her theorem. In reality, 1918’s Nobel (given in 1919 due to WWI) went to Max Planck for the discovery of energy quanta – we do not question Planck’s merit, but in our alternate timeline we add Noether as a co-laureate in 1918. This corrects a gender bias and highlights that theoretical contributions of the highest order were present in that era. It is fitting: Noether’s theorem is “arguably the most important result in theoretical physics”, yet she was unsung at the time. By honoring her, we acknowledge how far ahead of their time some contributions can be – requiring a later generation (and a merit-based perspective) to fully appreciate.
Satyendra Nath Bose (1894–1974) – Quantum Statistics Pioneer. Bose, an Indian physicist, in 1924 derived Planck’s law for blackbody radiation using novel statistical arguments, treating photons as indistinguishable particles – a radical idea then. He sent his paper to Einstein, who recognized its brilliance and translated it to German (adding some commentary of his own), which led to the concept of Bose-Einstein statistics and prediction of the Bose-Einstein Condensate (BEC), a new state of matter. Particles that follow Bose-Einstein statistics are now called bosons in his honor. Bose’s work was fundamental to quantum mechanics; it provided the first clear evidence that quantum particles do not behave like classical distinguishable objects. However, Bose was not awarded a Nobel Prize, even after quantum theory was established. Over the decades, at least seven Nobel Prizes in physics were awarded for research related to bosons, Bose-Einstein statistics, or BEC (for example, the 2001 Nobel for creating BEC in the lab). It is a striking historical irony that “although seven Nobel Prizes were awarded for research related to S.N. Bose’s concepts…, Bose himself was not awarded a Nobel Prize.”. Possible reasons include that Bose’s initial work was theoretical (and credited alongside Einstein), and later, by the time BEC was realized experimentally (1990s), Bose was long deceased. Our merit-based timeline insists on giving credit where due: we award S.N. Bose a Nobel Prize in Physics, circa 1924 (or jointly in the late 1920s) recognizing “his foundational work in quantum statistics and the prediction of new states of matter.” This could coincide with, say, the 1927 prize (in reality given to Compton and C.T.R. Wilson) or 1930 (given to Raman for light scattering – interestingly, another Indian physicist). In our expanded view, Bose stands tall as a direct intellectual progenitor of a whole sector of quantum physics, and it is only just that he receive the same honor as those who later applied his ideas.
Henrietta Swan Leavitt (1868–1921) – Measuring the Universe. Leavitt was an American astronomer whose 1908–1912 work on Cepheid variable stars established the period–luminosity relation, a breakthrough that allowed astronomers to measure distances to faraway galaxies. Her discovery directly enabled Edwin Hubble in 1920s to prove that the universe extends beyond the Milky Way and to discover cosmic expansion. While astronomy/cosmology was not a Nobel category, Hubble’s work received consideration for the Physics Nobel (though he died before he could be awarded). Leavitt, being a woman and merely an “assistant” at Harvard College Observatory, got little recognition in her life (she died of cancer in 1921). In a meritocratic retelling, Henrietta Leavitt’s contribution is Nobel-worthy for it provided the “standard candle” that made modern cosmology possible. We would envision a world where the Nobel Committee (had they been more forward-looking and less biased) might have created a special Nobel citation or included her in a prize on cosmological discoveries. For instance, if Hubble were to be honored, Leavitt’s role should be co-recognized. In our timeline, we note her achievement in the early 1920s and retroactively acknowledge her as a laureate-level contributor to physics (even if only through a hypothetical joint award). This highlights correction of both a gender bias and the interdisciplinary gap (astronomy vs physics).
2.3 Mid-20th Century – Recognizing the Unrecognized (Women, Teams, and Thinkers)
The mid-20th century saw the explosion of big physics (nuclear, particle, space) and the rise of large collaborations, as well as continued underrepresentation of certain groups. Here we identify some key overlooked contributors from roughly the 1930s to 1960s:
Lise Meitner (1878–1968) – The Forgotten Mother of Nuclear Fission. An Austrian-Swedish physicist, Meitner was part of the team that discovered nuclear fission in 1938–39. She provided the theoretical explanation for how a uranium nucleus could split and release enormous energy, working with her nephew Otto Frisch after she had fled Nazi Germany. Her longtime collaborator Otto Hahn conducted the chemical experiments in Berlin that identified the barium byproduct, evidence that the uranium had split. In 1944, the Nobel Prize in Chemistry was awarded solely to Hahn for the discovery of fission, pointedly excluding Meitner. Historians have pored over this decision: “for some 50 years after nuclear fission was discovered in 1938, Lise Meitner’s contributions … were not acknowledged”, and Nobel records (when later opened) showed she had been nominated multiple times but overlooked. The exclusion was likely a combination of factors – Meitner being a woman, Jewish (thus having to flee and not being there when the Nobel credit was assigned), and the Nobel committees of the time perhaps viewing the discovery as chiefly chemical (Hahn) even though physics was central. In our corrected timeline, Lise Meitner receives a Nobel Prize in Physics in 1944 (or is at least co-awarded a Nobel with Hahn, whether in Physics or Chemistry). We specifically note it in Physics to emphasize the physical theory she provided (as opposed to the chemical detection). Her citation: “for the discovery of nuclear fission, theoretical interpretation and understanding of its mechanisms.” This rightfully places Meitner alongside Marie Curie as female pioneers in nuclear science. The injustice of her omission has been widely recognized – she is now often cited as a prime example of an overlooked scientist in Nobel history. We rectify that, demonstrating that merit knows no gender or wartime politics.
Chien-Shiung Wu (1912–1997) – The First Lady of Physics. C.S. Wu was a Chinese-American experimental physicist who devised and carried out the critical experiment in 1956 that proved parity is not conserved in weak nuclear interactions. This overturned a fundamental “law” of physics (parity symmetry) that had been unquestioned for decades. The idea to test parity violation was proposed by theoreticians Tsung-Dao Lee and Chen Ning Yang, but it was Wu’s painstaking experiment using cobalt-60 that provided the definitive evidence. The discovery was announced in 1957 and caused a sensation. That same year, Lee and Yang received the Nobel Prize in Physics for the idea of parity violation, but Wu was not included. The Nobel committee, in its traditional pattern, awarded the theorists and neglected the experimentalist who confirmed the theory – ironically so, because without Wu’s result the theory would have remained speculation. By all accounts, this was a glaring oversight: Wu’s work was “termed the most important development in the field of atomic and nuclear physics to date” at the time. It was an open “secret” that she had been unfairly left out, likely due to her being a woman and perhaps the committee’s conservative approach. In a merit-only scenario, Chien-Shiung Wu is awarded the Nobel Prize in 1957 alongside Lee and Yang. In fact, one could argue all three should share it equally – theory and experiment together cracking a key symmetry of nature. Wu’s exclusion is now widely regarded as one of the great Nobel injustices. Our timeline rescinds that injustice, highlighting her as the example that experimental skill and insight is as valuable as theoretical – and that women in physics, no matter how brilliant, often faced barriers to recognition that we must consciously remove.
Jocelyn Bell Burnell (b. 1943) – Discoverer of Pulsars. In 1967, as a graduate student, Jocelyn Bell (now Dame Jocelyn Bell Burnell) discovered the first pulsating radio sources (pulsars) through meticulous observation of radio telescope data. This was a monumental discovery – pulsars were later understood to be rotating neutron stars, an entirely new type of astrophysical object, providing confirmation of theoretical predictions of neutron stars and opening a new field of research. However, the Nobel Prize in Physics 1974 for the discovery of pulsars was awarded to her PhD supervisor, Antony Hewish (and jointly to radio-astronomer Martin Ryle), omitting Bell. This caused an outcry in the scientific community; many felt Bell, as the actual observer who noticed the anomalous signal, should have been included. The Nobel committee’s rationale seemed to be that as a student, her contribution was part of her advisor’s project – an attitude now widely criticized as sexist and hierarchical bias. Bell Burnell herself handled the snub with grace and went on to a distinguished career, and in 2018 she was recognized with the $3 million Special Breakthrough Prize in Fundamental Physics, explicitly acknowledging that she had been “overlooked by the Nobels”. In our corrected timeline, Jocelyn Bell Burnell receives the Nobel Prize in Physics in 1974 jointly with Hewish (and Ryle). If only three can win, we include her and perhaps omit one of the others, but since our rules allow more than three, all contributors can be named. The merit is clear: without her diligence, the pulsar discovery might have been missed or delayed – she literally saw what others did not. Recognizing her also underscores a broader point: that the contributions of students, junior researchers, and especially women have historically been downplayed, something a fair system must fix.
Alan Turing (1912–1954) and Claude Shannon (1916–2001) – Founders of Information Theory and Computing. Neither Turing nor Shannon worked strictly as “physicists”, yet their work has had profound implications for physical sciences and the modern world. We include them as interdisciplinary geniuses whose contributions meet our criterion of fundamental, long-term impact:
- Alan Turing: British mathematician, logician, and cryptanalyst, often dubbed the father of theoretical computer science and artificial intelligence. In 1936, he developed the concept of the Turing machine, providing a formal definition of computability and the modern notion of an algorithm. He also was pivotal in World War II codebreaking (Enigma), and after the war, he delved into early AI concepts and even mathematical biology. There is no Nobel Prize for mathematics or computing, but the ACM’s Turing Award (established 1966) is named in his honor and is considered the “Nobel Prize of Computing”. The fact that computing had to create its own Nobel equivalent, named after Turing, says much: “The A.M. Turing Award, sometimes referred to as the ‘Nobel Prize in Computing,’ was named in honor of Alan Turing… [who] made fundamental advances in computer architecture, algorithms, formalization of computing, and AI.”. In our expanded Nobel landscape, we envisage a joint award in the mid-20th century for the theoretical foundations of information and computation. Perhaps around 1950 (had history been different), Turing and Shannon could have shared a Nobel Prize in Physics or a hypothetical “Information Science” category. Turing died in 1954 under tragic circumstances, so realistically he never got that chance. In merit terms, we acknowledge Alan Turing for “formulating the mathematical foundations of computation and artificial intelligence”. His legacy in shaping computational theory is comparable to a new law of nature in its importance for the human future.
- Claude Shannon: An American mathematician and electrical engineer, Shannon in 1948 published “A Mathematical Theory of Communication,” which founded the field of information theory. He defined the bit, introduced entropy in information context analogously to thermodynamic entropy, and established the limits of data compression and reliable communication over noisy channels (Shannon’s Theorem). This work underpins all modern digital communications – the internet, telecommunications, data storage – earning Shannon titles like “father of the information age”. Like Turing, Shannon didn’t receive a Nobel because none exists for his field. But he did receive high honors (National Medal of Science, Kyoto Prize). It’s often noted that “Shannon worked in a field for which no Nobel prize is offered.” (Indeed, an IEEE society quote goes: “While Shannon worked in a field for which no Nobel prize is offered, his work was richly rewarded by honors…”). In a merit-centric view that doesn’t silo disciplines, Claude Shannon merits recognition equivalent to a Nobel for “establishing the mathematical foundation of information theory,” which has deep connections to physics (e.g., in thermodynamics and quantum information). Our alternative history imagines Shannon sharing that hypothetical mid-century prize with Turing. By including Shannon and Turing, we emphasize that the conceptual revolutions in how we process information and compute are as fundamental to humanity’s scientific trajectory as any discovery in physical law.
Teams and Collaborations: By mid-century, big projects like the Manhattan Project (development of nuclear reactors and weapons), large particle accelerators, and international observatories became common. The Nobel system, focusing on at most three individuals, inherently under-recognized these team efforts. Two illustrative cases:
- Manhattan Project (1940s): This was a massive joint effort of scientists (Fermi, Oppenheimer, Szilard, Teller, etc.) that led to the first sustained nuclear chain reaction (Chicago Pile, 1942) and the first atomic bombs (1945). The Nobel Committee did honor some individuals – e.g., Enrico Fermi won the 1938 Nobel for inducing radioactivity (just before he joined the project), and Emilio Segrè and Owen Chamberlain later won for discovering the antiproton (1959) which was partly post-war physics. But key figures like J. Robert Oppenheimer (scientific director of Los Alamos) never received a Nobel – partly because his direct scientific output was diffuse (he was more of a leader than one who made a singular discovery). In our merit review, we argue that certain achievements attributable to large teams could be recognized through special Nobel citations or prizes to collaborations. For example, the achievement of controlled nuclear chain reaction could have been cited in a Nobel Prize to Fermi and collaborators in 1944 (though wartime secrecy would have prevented it then; but retrospectively we mark it). We include this discussion to show that merit-only recognition might require breaking the traditional mold of individual awards and sometimes say “this year’s Nobel Prize honors the X Collaboration for…”. Though Nobel rules didn’t allow it, in a perfect meritocracy it might.
- Particle Physics Collaborations (1960s and beyond): Experiments discovering new particles (quarks, W/Z bosons, Higgs boson, etc.) often involved hundreds or thousands of scientists. Historically, a few leaders or representatives get the Nobel. For instance, the 2013 Nobel in Physics to Peter Higgs and François Englert for the Higgs mechanism left out four other theorists who independently co-developed the theory as well as the entire CERN experimental teams of ATLAS and CMS who found the particle. Our timeline in 2012/2013 would list all the key theorists (including Anderson, Guralnik, Hagen, Kibble, ’t Hooft – noting Brout died in 2011) and at least symbolically the ATLAS/CMS collaborations or their spokespeople. Similarly, in 2002 the Nobel for neutrino oscillations went to two leaders (Koshiba and Davis) but not the many others in Super-Kamiokande or SNO experiments; 2017 gravitational wave detection Nobel went to three individuals (Weiss, Barish, Thorne) and not the 1000+ LIGO team – we would broaden those. While it’s impractical to list every name, a merit-based ethos would at least say “prize to X, Y, and the Z collaboration”. We will incorporate this approach in Chapter 3’s timeline narrative.
By highlighting these cases in the mid-century, we see patterns: women scientists systematically overlooked (Meitner, Wu, Bell), non-Western scientists undercredited (Bose), theorists underappreciated until too late (Noether, arguably also John von Neumann could be mentioned as an early computer pioneer – though he also had a broad impact in many fields), and large group efforts recognized only in piecemeal fashion. The remainder of the 20th century continued these trends to an extent, though there was gradual improvement in some areas (e.g., by late 20th century, theorists like Weinberg/Salam/Glashow did get recognized promptly for unification theory in 1979; but still others like Stephen Hawking never got Nobel because their predictions took too long to verify or were not yet verified before death).
2.4 Late 20th Century and Beyond – Emerging Fields and Today’s Overlooked
In the latter part of the 20th century and early 21st century, the Nobel Prize in Physics diversified somewhat (with awards in astrophysics, quantum phenomena, etc.), but some contributors and areas remain undervalued:
Stephen Hawking (1942–2018) – Hawking, a theoretical physicist, made seminal contributions to cosmology, gravity, and quantum theory (especially the prediction of Hawking radiation from black holes). Though globally famous, he never won a Nobel Prize, because his key prediction lacked experimental confirmation until perhaps very recently (and even now, detecting Hawking radiation directly is extremely challenging). Hawking’s case exemplifies the Nobel committee’s reluctance to award unproven theory. In a merit-based retrospective, one could argue Hawking’s synthesis of general relativity and quantum mechanics in the context of black holes was so influential that it deserved recognition even absent direct proof (or one could say the “edge of proof” – like the indirect evidence via analog systems, etc.). Our timeline might consider a special Nobel in the 1980s acknowledging the theoretical strides in cosmology (perhaps shared with others like Roger Penrose, who eventually did get a Nobel in 2020 for related work, and Yakov Zeldovich posthumously, etc.). We mention Hawking as a representative of speculative but profound theoretical work that a purely merit-focused culture might still honor for its intellectual daring and impact on the field, even if empirical confirmation lags.
Experimentalists behind precision tests: For instance, Joseph Weber, who first attempted to detect gravitational waves in the 1960s – he claimed detections that were later not confirmed, so he did not get a Nobel and is often seen as wrong. However, his pioneering efforts kicked off a whole field leading to LIGO’s success decades later. In a sense, merit-only history might acknowledge trailblazers even if they personally didn’t achieve the final result, because their contribution was essential. This is a nuanced point – generally Nobels don’t reward failed attempts, but a holistic view of progress might.
Unknown Unknowns of Today: We consider if there are scientists or fields currently under the radar that future generations will regard as Nobel-caliber. This is inherently speculative. Some candidates:
- Quantum Foundations/Quantum Computing: Individuals like John Bell (1928–1990) who formulated Bell’s theorem on quantum entanglement. Bell’s work was finally vindicated by experiments long after his death, and indeed the 2022 Nobel in Physics went to Aspect, Clauser, and Zeilinger for experimental tests of Bell’s inequalities. Bell did not live to see that Nobel; in our timeline we might posthumously acknowledge John Bell’s profound insight as deserving of a Nobel in the 1980s had the experiments been done earlier. Similarly, early quantum computing theorists (David Deutsch, Peter Shor) have not been recognized yet because practical quantum computers are still emerging. But their contributions might be seen as Nobel-worthy in time.
- Complex Systems and Chaos: People like Ilya Prigogine (Nobel in Chemistry 1977 for non-equilibrium thermodynamics) did get recognized, but others like Edward Lorenz (chaos theory pioneer in meteorology) did not since there’s no Nobel for mathematics or meteorology. Yet chaos theory now permeates physics. A merit-based view might include chaos theory founders in the story of physics breakthroughs.
- Interdisciplinary fields that might be undervalued: e.g., biophysics and neuroscience from a physics perspective. If one views the brain or life as physical systems, contributions at this interface might someday be seen as fundamental. For instance, the physics of complex networks – scientists like Duncan Watts or Albert-László Barabási who studied network theory, or Giorgio Parisi who worked on complex systems (Parisi did win in 2021 for spin glasses and complex systems). Maybe the broader idea is that some pioneering work in understanding complexity and emergence could be an “unknown unknown” that in the future is regarded as fundamental.
- Climate and Geophysics: The 2021 Nobel in Physics recognized climate modeling (Manabe and Hasselmann) – a sign that even traditionally “applied” fields are now seen as fundamental physics. But earlier contributors to chaos and climate, like Lorenz mentioned, were not honored in their time.
We raise these points to show that even today, merit-based recognition is a moving target – our understanding of what is significant can evolve. Thus, the list of overlooked contributors is never final; it reflects the state of knowledge and values of each era. The purpose of our exercise is to anticipate and correct for those shifts in hindsight.
Having profiled many individuals and groups that deserve greater acclaim, we will now proceed to Chapter 3, where we integrate these insights into a cohesive alternative Nobel Prize timeline. There, year by year (or decade by decade), we will enumerate the Nobel awards as they actually happened and contrast them with how they would happen under our merit-only criteria. This will concretely show the additions, omissions, and changes we are proposing – effectively a “re-awarding” of over a century of Nobel Prizes in Physics.
Chapter 3: A Revised Nobel Prize Timeline (1901–2023)
In this chapter, we present the year-by-year alternative Nobel timeline informed by our merit-only analysis. To make this comprehensive yet digestible, we divide the timeline into eras, highlighting key adjustments and providing representative examples. A full detailed listing, including every year’s laureates as originally awarded and our proposed changes, is available in Appendix A (Comparative Nobel Laureate Table). Here, we summarize and discuss the most significant revisions.
3.1 Pre-Nobel Era Recognitions (1680s–1899)
While the Nobel Prizes began in 1901, our merit-based history issues retroactive “Nobel Prize” honors to seminal contributors in earlier centuries (as previewed in Chapter 2). These do not correspond to actual Nobel years but serve as a prologue of foundational achievements:
- 1687 (Retroactive): Isaac Newton – for “universal gravitation, laws of motion, and the mathematical invention of calculus”. (Had a Nobel Prize existed, Newton’s Principia Mathematica would have been the quintessential award-winning work in late 17th century.)
- 1750 (Retroactive): Leonhard Euler – for “analytical mechanics and mathematical physics foundations”.
- 1788 (Retroactive): J.L. Lagrange – for “Lagrangian mechanics, reformulating the laws of nature via least action”.
- 1830s (Retroactive): Carl F. Gauss – for “electromagnetism (Gauss’s law), precision measurements, and mathematical tools for physics”.
- 1840 (Retroactive): Michael Faraday – for “experimental discoveries in electromagnetism (induction, etc.) and electrochemistry”.
- 1854 (Retroactive): Bernhard Riemann – for “development of differential geometry, enabling later theories of spacetime”.
- 1865 (Retroactive): James Clerk Maxwell – for “unification of electricity, magnetism, and light; and contributions to statistical physics”.
- 1865 (Retroactive, same year): Gregor Mendel – for “pioneering statistical laws of inheritance, effectively applying quantitative physics thinking to biology”. This one is particularly interesting: we mark Mendel’s 1865 work (though he was a biologist/monk) as an example of cross-disciplinary merit – in our view, his rigorous use of statistics in natural science was as foundational as any 19th-century physics discovery.
- 1870s–1880s (Retroactive): Ludwig Boltzmann and J. Willard Gibbs – for “founding statistical mechanics”. We might assign Boltzmann to 1905 (the year we think he deserved the Nobel had he been alive, and also symbolically when Einstein confirmed atomism), and Gibbs to 1902 (since he died 1903, this is when he could have been honored).
- 1890s (Retroactive): Heinrich Hertz (discovering radio waves, confirming Maxwell’s theory, ~1887), J.J. Thomson (electron discovery, 1897 – though he actually got the 1906 Nobel), Nicholas Tesla and Guglielmo Marconi (radio technology – Marconi did get 1909 Nobel, Tesla did not; we might include Tesla as co-laureate retroactively for radio or AC electrical systems). Also Dmitri Mendeleev (periodic table, 1869 – more chemistry, but fundamental order of elements; he was nominated for Nobel in Chemistry but never won). We won’t list everyone, but these are noted in Appendix.
This retroactive list establishes that by 1901, our timeline already acknowledges a pantheon of giants who set the stage. In real history, early Nobel committees often gave prizes to slightly lesser figures because the greatest (Newton, etc.) were long gone. Our approach instead pays homage to those giants formally.
3.2 1901–1913: The Classical Nobel Beginnings Revisited
The first Nobel Prizes in Physics (1901 onward) in actual history went to a series of groundbreaking discoveries, many of which we agree with. We preserve most of the original early laureates, while adding some names:
- 1901: Wilhelm Conrad Röntgen – (discovery of X-rays) – Retain. This was the inaugural Nobel in Physics and clearly merit-based. X-rays revolutionized both physics and medicine.
- 1902: Hendrik Lorentz and Pieter Zeeman – (Zeeman effect and electron theory) – Retain. We agree; Lorentz’s electron theory and Zeeman’s splitting of spectral lines under magnetism were fundamental.
- 1903: Antoine Henri Becquerel, Marie Curie, Pierre Curie – (discovery of radioactivity) – Retain. We affirm the joint award, perhaps emphasizing Marie Curie’s role even more (she went on to win another Nobel in 1911 in Chemistry).
- 1904: Lord Rayleigh (John Strutt) – (discovery of argon, properties of gases) – Retain, though this is borderline (it’s chemistry/physics intersection). It was given, we accept it as merit (for precision measurements).
- 1905: Philipp Lenard – (cathode rays) – Modify. In reality Lenard won for work on cathode rays leading to electron physics. However, Lenard’s contribution, while notable, was not singular (Thomson had discovered the electron itself by 1897). Moreover, Lenard later became a proponent of Nazi “Deutsche Physik” and an opponent of relativity – not that this affects the merit of his early work, but historically it tarnishes his legacy. We would consider either not awarding Lenard in 1905, or awarding him jointly with J.J. Thomson (who won in 1906). Alternatively, we might say Ludwig Boltzmann should have been honored in 1905 instead. Indeed, Boltzmann’s death in 1906 makes 1905 the last chance; by 1905 his statistical mechanics was finally gaining evidence (Einstein’s papers on Brownian motion gave empirical validation to atomic theory, a posthumous vindication for Boltzmann). So in our timeline: 1905 Nobel in Physics to Ludwig Boltzmann (for statistical mechanics), possibly alongside someone like Thomson or Lenard if necessary. We thus mark Boltzmann’s much deserved recognition at this point.
- 1906: J.J. Thomson – (discovery of the electron) – Retain (if not already honored in 1905). Thomson’s discovery was fundamental, we keep him, possibly shifting him to 1905 if we combine with Lenard.
- 1907: Albert A. Michelson – (optical precision instruments, Michelson-Morley experiment) – Retain. Michelson’s work was crucial in establishing the constancy of the speed of light (his null result paved way for relativity). We definitely keep him.
- 1908: Gabriel Lippmann – (color photographic method) – Debatable. Lippmann’s invention was clever but arguably not as impactful as others. However, 1908 didn’t have an obvious overlooked physicist waiting. One might consider Guglielmo Marconi had not yet been honored (Marconi gets 1909) or Heike Kamerlingh Onnes (who made big strides in 1908 by liquefying helium, but he got 1913). We might simply accept Lippmann but note that “in an alternate view, the 1908 prize might have been awarded to X”. For now, we’ll keep Lippmann but mark it as one of the less imperative awards.
- 1909: Guglielmo Marconi and Karl Ferdinand Braun – (wireless telegraphy) – Retain, but Add Nikola Tesla. The 1909 prize credited Marconi and Braun for radio technology. Many argue Nikola Tesla (who demonstrated radio transmission before Marconi in some forms and held patents that Marconi’s work infringed) deserved co-credit. In our timeline, we would include Nikola Tesla as a co-laureate in 1909 for his contributions to wireless communication and electrical engineering. Since Nobel rules allow three, this fits. Tesla was indeed nominated for the Nobel in that era and is a famous omission. So 1909 becomes Marconi, Braun, and Tesla all recognized for making radio a reality.
- 1910: Johannes Diderik van der Waals – (equation of state for gases and liquids) – Retain. His equation was foundational in understanding phase transitions; merit is strong.
- 1911: Wilhelm Wien – (Wien’s displacement law for blackbody radiation) – Retain, albeit with context. By 1911, Planck’s law had superseded Wien’s as a full explanation of blackbody spectra, but Wien’s earlier empirical law was key evidence for quantum theory. We keep it, noting perhaps that Planck would soon be honored for the deeper theory.
- 1912: N/A – No prize awarded. Historically, 1912 was skipped for Physics (the committee didn’t find a suitable awardee in time). In our timeline, 1912 Nobel Prize in Physics goes to Henri Poincaré (posthumously, since he died July 1912, but our rules allow that) or ideally should have been given while he was alive. Given Poincaré had 34 nominations in 1910 alone, it is plausible to imagine him being awarded in 1912 had the committee resolved their hesitations. We assign 1912: Henri Poincaré – “for his numerous contributions to mathematical physics, including the theory of relativity and the three-body problem.” This single addition corrects one of history’s biggest omissions. If needing to adhere to no-posthumous, one could argue he should have gotten 1910 or 1911, but since 1912 was empty, it’s poetic to fill the gap with his name.
- 1913: Heike Kamerlingh Onnes – (low-temperature physics, superconductivity discovery) – Retain. Onnes liquefied helium in 1908 and discovered superconductivity in 1911 – absolutely a Nobel-worthy achievement. We keep him as is.
In addition, 1913 in our timeline also sees a special recognition: Niels Bohr for his atomic model. Historically, Bohr got the 1922 Nobel for his 1913 model of the hydrogen atom. In our timeline we might expedite that: Bohr’s model was proposed in 1913, and by 1914–1915 it was widely celebrated. However, due to WWI, Nobels around that time were delayed. Bohr’s prize being given in 1922 is fine, but we’ll leave it as is for 1922 in consistency with actual. (This raises an approach: do we strictly keep actual year ordering for things that did get recognized? Generally yes, except where we feel it was undeserved or there was an overlooked co-laureate.)
Summary of 1901–1913 changes: We mostly align with actual awards but add Boltzmann (1905), Tesla (1909), Poincaré (1912) and possibly adjust Lenard’s role in 1905. By 1913, our alternate Nobel list has a richer set of laureates, including mathematicians and inventors who in reality got no Nobel.
3.3 1914–1929: Relativity, Quantum, and the Changing World
The period of World War I and the 1920s was transformative for physics (relativity confirmed, quantum mechanics born). The Nobel history during this time had to catch up with radical theory while dealing with war and its aftermath. Here’s how our timeline unfolds:
- 1914: Max von Laue – (X-ray diffraction in crystals) – Retain. This experiment showed X-rays (electromagnetic waves) diffract like light, confirming their wave nature and giving birth to X-ray crystallography. We keep von Laue.
- 1915: William Henry Bragg and William Lawrence Bragg – (X-ray crystallography) – Retain. Father-and-son team who used Laue’s idea to determine crystal structures (they essentially founded modern crystallography). Definitely merit-based.
- 1916: No award (WWI) – Modify: Perhaps if war had not intervened, 1916 could have honored someone like Einstein for general relativity (published 1915). However, relativity’s confirmation only came in 1919’s eclipse results. The Nobel committee was cautious and did not honor Einstein for relativity at that time. In our timeline, since we allow a bit more boldness, we might consider 1916: Albert Einstein for Special and General Relativity. But Einstein was still alive and would get recognized later anyway. We might leave 1916 empty due to war, and plan to honor Einstein soon after confirmation.
- 1917: No award (WWI) – No change, war disrupted science.
- 1918: Max Planck – (quantum hypothesis) – Retain Planck, but Add Emmy Noether. As discussed, we include Emmy Noether (1918) for her symmetry/conservation theorem. Historically, Planck’s 1918 prize recognized his 1900 quantum leap; we still give Planck his due. We consider this year to have two laureates: Planck and Noether. (Original Nobel rules allow 2 laureates in a year easily.)
- 1919: Johannes Stark – (Stark effect, splitting of spectral lines in E-field) – Question. Stark’s award is controversial; while the Stark effect was a real discovery, Stark was politically favored as a German patriotic pick after WWI (where Germany had been isolated). Stark also later became a prominent Nazi sympathizer. Even purely scientifically, the Stark effect, though important, could be seen as a minor prize compared to those who were still not awarded (like Einstein at that time). In our timeline, 1919 would not be awarded to Stark. Instead, 1919 might honor Ernest Rutherford. Rutherford did not get a Physics Nobel (he got the 1908 Chemistry Nobel for his earlier work on radioactivity) – but in 1919 he discovered the proton (by knocking hydrogen nuclei out of nitrogen). Perhaps more fitting: 1919: Arthur Eddington and Albert Einstein – for the empirical confirmation of general relativity (Eddington’s eclipse expedition). However, awarding Einstein in 1919 could be tricky as a war-traitor in some eyes. Alternatively, skip 1919 or pick Rutherford for discovering the proton (though he was already a laureate via chemistry). Let’s propose: 1919: Albert Einstein (finally) for his body of work including relativity and photoelectric effect. In reality, Einstein was not awarded until 1922 (delayed Nobel for 1921). We can accelerate that to 1919 in our idealized scenario, especially given the dramatic 1919 proof of starlight bending. If we do that, then Einstein’s later 1921 Nobel slot is freed up to acknowledge something else. We might do this because Einstein truly deserved multiple Nobels (he had nominations every year in 1910s). By awarding him now for relativity, we correct the “hesitancy” problem. We also remove Stark (whose merit was comparatively smaller and tainted by politics). So: 1919: Einstein (relativity, etc.) instead of Stark.
- 1920: Charles Édouard Guillaume – (precision metrology, Invar alloy) – Debatable. Guillaume’s Nobel for discovering a low-expansion alloy (useful for clocks etc.) was seen as a somewhat applied award. In a pure merit timeline, 1920 might be better used. Candidates: Niels Bohr (who published his model in 1913 and by now had strong support), H.A. Lorentz (though already Nobel ’02, but his relativity contributions?), Arnold Sommerfeld (a great atomic theorist, never won Nobel), or Theoretical pioneers like Boltzmann or Gibbs had they not been recognized yet (but we did them earlier). We could use 1920 to honor Niels Bohr if we hadn’t already. Bohr in fact got 1922 Nobel (for 1921). Alternatively, 1920: Arnold Sommerfeld might be recognized for his contributions to atomic theory and mentoring a generation (but Nobel rarely awards mentorship or breadth). Another approach: use 1920 to honor the development of quantum theory collectively – maybe a joint prize to Bohr, Arnold Sommerfeld, and Max Born (the latter did get a Nobel in 1954 eventually). This is speculative. Let’s simplify: 1920: Niels Bohr (since we gave Einstein earlier, Bohr can slide here instead of 1922). We then might skip Guillaume, or make him a footnote; his work, while valuable, doesn’t match Bohr’s in impact.
- 1921: (Nobel not awarded in 1921, later used for Einstein in 1922) – In our scenario, since Einstein is already awarded, 1921 can be used for someone else. We propose 1921: Ernest Rutherford (Physics) for “discovering the atomic nucleus and proton, and for pioneering nuclear physics.” Rutherford’s 1908 Nobel in Chemistry left a gap that his later nuclear scattering work (like the 1911 nucleus, 1919 proton discovery) wasn’t honored by Physics Nobel. So 1921 is a good spot to recognize Rutherford in physics as well. Alternatively, 1921 could honor H.G.J. Moseley (who discovered the atomic number concept via X-ray spectra in 1913, but died in WWI in 1915) – Moseley’s work was so important that many felt he would have won a Nobel had he lived. We allow posthumous, so we could posthumously honor Moseley around 1921 for establishing the concept of atomic number (a key to the periodic table). However, Rutherford’s contributions by 1921 were more widely celebrated. Possibly we can do a split: 1921: Rutherford and Moseley (Moseley posthumously) to emphasize nuclear atom and atomic number. That would be a nice correction of war’s toll on science (Moseley’s death at 27 was a great loss).
- 1922: Albert Einstein (for 1921) – (photoelectric effect) – In actual history, Einstein’s Nobel was given in the 1922 ceremony, citing the photoelectric effect and “services to theoretical physics” (but not relativity). In our timeline, Einstein is already laureate. So 1922 is freed up. Historically, Niels Bohr received the 1922 Nobel (for his 1913 atomic model). We gave Bohr the 1920 slot, so who now? Possibly 1922: Arnold Sommerfeld and Max Born – recognizing their roles in advancing quantum theory (Sommerfeld’s fine-structure constant work, elliptical orbits; Born’s statistical interpretation of wavefunction came later by 1926). Sommerfeld was nominated many times but never got Nobel; Born eventually did in 1954 for a later piece. Or we could use 1922 to honor the Stern-Gerlach experiment (1922) which demonstrated space quantization of atomic spins, a key early quantum experiment. Otto Stern and Walther Gerlach’s result confirmed aspects of quantum theory experimentally. Historically Stern got a Nobel in 1943 (Gerlach did not). We might move that up: 1922: Otto Stern and Walther Gerlach for the Stern-Gerlach experiment. This acknowledges an important experiment at its time. Let’s adopt that: 1922: Stern & Gerlach – “for the experimental demonstration of quantization (Stern-Gerlach experiment)”.
- 1923: Robert Millikan – (measurement of electron charge, photoelectric effect verification) – Retain. Millikan’s oil-drop experiment and his work verifying Einstein’s photoelectric equation were indeed Nobel-worthy. We keep him.
- 1924: Manne Siegbahn – (X-ray spectroscopy) – Retain. Important contributions to precision X-ray spectroscopy, enabling atomic research, seems fine.
- 1925: James Franck & Gustav Hertz – (Franck-Hertz experiment proving quantized atomic energy levels) – Retain. Their 1914 experiment was a crucial confirmation of Bohr’s theory; absolutely deserving.
- 1926: Jean Baptiste Perrin – (confirmation of Brownian motion, Avogadro’s number) – Retain. Perrin’s work confirmed atomistic theory (e.g., verifying Einstein’s Brownian motion predictions), which was hugely important for establishing molecular reality – indeed a follow-up of Boltzmann/Einstein. We keep Perrin.
- 1927: Arthur Compton & C.T.R. Wilson – (Compton effect; cloud chamber) – Retain. Both of these are solid: Compton’s experiment showed photon particle nature; Wilson invented the cloud chamber for particle detection. Good picks.
- 1928: Owen Richardson – (thermionic emission law) – This is a lower-profile award. Richardson’s law for electron emission was useful, but in a merit re-check, 1928 could be repurposed. Who might we have missed by 1928? S.N. Bose and M.S. Chandrasekhar come to mind. Bose’s work was 1924; Chandrasekhar calculated the white dwarf limit in 1930 (so that’s later). Satyendra Nath Bose could be honored around this period: maybe 1928: S.N. Bose (and perhaps also Fermi who independently did Fermi-Dirac stats in 1926) for quantum statistics. Enrico Fermi would later get 1938 Nobel for neutron stuff, but his 1926 formulation of Fermi statistics was also fundamental. We could do 1928: S.N. Bose and Enrico Fermi – “for statistical theories of quantum systems (Bose-Einstein and Fermi-Dirac statistics)”. This would be a neat recognition of theory that later proved incredibly impactful (and a symmetry: bosons and fermions both covered). Historically, no such award was given (Fermi’s Nobel was for something else; Bose got nothing). We like this change, as it acknowledges Bose’s contribution in a timely fashion and gives Fermi an early accolade for his theoretical contribution (Fermi did so much we won’t mind giving him two Nobels in different contexts; our rules allow it).
- 1929: Louis de Broglie – (wave nature of electrons) – Retain. His matter-wave hypothesis was revolutionary for quantum mechanics; absolutely keep.
By 1929, our timeline has integrated many key theoretical contributions earlier (Einstein earlier, Bose recognized, Noether in 1918, Poincaré recognized, etc.) compared to actual history, which sometimes waited or never awarded them. We have also ensured a couple of deserving experimentalists (Tesla, Stern-Gerlach team) got their due.
3.4 1930–1945: Quantum Mechanics and Nuclear Physics – New Stars and Missed Chances
The 1930s saw the full development of quantum mechanics and the advent of nuclear/particle physics. The Nobel awards in this era largely went to the founders of quantum theory and early nuclear discoveries. We will mostly follow but make crucial additions:
- 1930: C.V. Raman – (Raman effect, light scattering) – Retain. Raman’s discovery of inelastic light scattering (Raman effect) was highly significant in spectroscopy. We keep him (and note he was the first non-Western science Nobel laureate in physics).
- 1931: (no award, reserved for later use) – Historically, 1931 was not awarded and later assigned to 1932’s presentation.
- 1932: Werner Heisenberg – (creation of quantum mechanics, Uncertainty Principle) – Retain. Heisenberg’s matrix mechanics and uncertainty principle were indeed deserving. However, historically this 1932 award was effectively given in 1933 alongside others.
- 1933: Erwin Schrödinger & Paul A.M. Dirac – (wave mechanics; Dirac equation & quantum theory) – Retain. They shared the 1933 prize and we fully agree; they were architects of quantum mechanics.
- Notably, Max Born did not get a Nobel at this time (he got one in 1954 for statistical interpretation). In our timeline, one could argue Born should have been included in 1933 as well, making three laureates (Heisenberg, Schrödinger, Dirac, Born – that’s four which is too many for one year unless we break rules). Instead, we might try to fit Born elsewhere earlier or later. Perhaps we can retroactively include Born as a co-laureate in 1932 with Heisenberg (since Born was Heisenberg’s mentor and co-developed matrix mechanics formalism)? Or in 1933 with Dirac & Schrödinger (making 3 laureates, which is allowed). Let’s do: 1933: Schrödinger, Dirac, and Max Born – to cover wave mechanics, relativistic QM, and statistical interpretation. Heisenberg 1932 alone is fine. This inclusion gives Born timely credit rather than waiting until 1954.
- 1934: no physics prize awarded. We could utilize 1934 for someone: options include Enrico Fermi (who by 1934 had done pioneering work on beta decay theory and neutron experiments), S.N. Bose if not yet awarded (but we gave him 1928), Chandrasekhar (Chandrasekhar was formulating his limit for stellar mass ~1930 and faced opposition from Eddington; he wouldn’t be recognized until much later, 1983 Nobel in astrophysics). Possibly 1934: Enrico Fermi for “creation of artificial radioactivity via neutron bombardment”. Historically Fermi got 1938 after discovering neutron-induced radioactivity and a hint of transuranic (which turned out to be fission). But by 1934 he’d done a lot. Let’s leave 1934 for later or skip.
- 1935: James Chadwick – (discovery of the neutron) – Retain. Fundamental discovery, we keep it.
- 1936: Victor Hess & Carl D. Anderson – (discovery of cosmic rays; discovery of positron) – Retain. Both cosmic rays and the positron were major discoveries. We keep them. Possibly we add Dmitri Skobeltsyn or others who nearly discovered positron earlier, but Anderson is fine.
- 1937: Clinton Davisson & George Paget Thomson – (electron diffraction) – Retain. Their experiments confirmed de Broglie’s wave hypothesis by showing electrons behave as waves. Absolutely merit.
- 1938: Enrico Fermi – (induced radioactivity via neutrons, discovery of transuranic?) – Retain, but note if we gave Fermi an earlier prize (for statistics or so), he’d now be a two-time laureate. That is acceptable; John Bardeen got two. So Fermi’s 1938 prize for experimental nuclear work stands even if he also had a 1928 for theory in our timeline. Fermi’s contributions were so extensive that multiple Nobels reflect that (as per our rule that if merit deserves multiple, so be it).
- 1939: Ernest Lawrence – (invention of the cyclotron) – Retain. The cyclotron was a game-changer in experimental physics, keep it.
- 1940–1942: no awards (WWII).
- 1943: Otto Stern – (molecular beam experiments, magnetic moment of proton) – Retain, albeit in our timeline Stern was already honored in 1922 with Gerlach for Stern-Gerlach. If we did that, Stern wouldn’t need another in 1943. Alternatively, 1943 actual was basically recognizing Stern’s lifelong contributions since war delayed any other picks. If Stern is already laureate, we can use 1943 to acknowledge another. Perhaps Maxwell’s demon concept? Probably skip reassigning 1943; Stern is fine either in 1922 or 1943 but not both. We can consider 1943 as open for an alternate pick if Stern was earlier: in that case, 1943 could go to Chandrasekhar (Chandrasekhar’s work on stellar structure in the 30s was finally being recognized; he actually got Nobel in 1983 for that). Or George Gamow (for alpha decay theory and big bang nucleosynthesis ideas, though that came later in ’40s). Or Michael Faraday if we hadn’t done retroactive (but we did). Let’s tentatively say 1943: Subrahmanyan Chandrasekhar for astrophysics (posthumous or not, he was alive though not recognized yet due to prejudices).
- 1944: Isidor Rabi – (discovery of NMR, resonance method) – Retain. Rabi’s molecular beam method and magnetic resonance are indeed fundamental, keep it.
- 1945: Wolfgang Pauli – (exclusion principle) – Retain. Pauli’s exclusion principle (1925) is a bedrock of atomic physics, he definitely deserved it (though he only got it in ’45, likely delayed by war).
Now crucial mid-40s note:
- 1944 (Chemistry Nobel) went to Otto Hahn for fission, excluding Meitner. In our timeline, we have:
- Lise Meitner getting a Physics Nobel in 1944 (maybe the same year as Rabi, or as a separate recognition in parallel). Possibly we say: 1944 Nobel Prize in Physics: Lise Meitner and Otto Frisch for nuclear fission theory, while Hahn could keep the Chemistry Nobel for the chemical discovery aspect. This ensures Meitner is honored. Alternatively, make Hahn share the chemistry Nobel with Meitner (but we’re focusing on Physics prizes in our analysis, so we give Meitner a Physics Nobel independently).
- Historically, no Physics prize was awarded in 1944 (Rabi’s was technically ’44 but given in ’45 due to war). Actually, correct: Rabi was announced in 1944. So we can do Rabi and Meitner both in 1944 by our extended share policy.
- 1945 Physics historically went to Pauli, which we keep. Another oversight: Chien-Shiung Wu’s parity experiment happened in 1956, so not yet relevant in this timeline portion.
By 1945, we have accounted for most major quantum and nuclear pioneers:
We included Bose, Noether, Poincaré, etc., who were missed, and fixed Meitner’s omission. We also possibly inserted Chandrasekhar earlier (four decades before his actual Nobel) – which is interesting and arguable; maybe we shouldn’t move him because his work’s significance wasn’t widely accepted until much later. But in a merit sense, his calculation was correct and fundamental. We can leave that as a speculative change.
3.5 1946–1960: Post-war Physics – Expanding the Particle Zoo and Fundamental Theory
After WWII, physics entered the era of particle physics (many new particles found), solid state physics, and early space science. The Nobel prizes diversified accordingly. We continue aligning mostly with actual awards, adding corrections:
- 1946: Percy W. Bridgman – (high-pressure physics) – Retain. Bridgman’s work on high-pressure experiments was foundational in material physics.
- 1947: Edward Appleton – (ionosphere physics) – Retain. Fundamental radio wave propagation discovery.
- 1948: Patrick Blackett – (cloud chamber developments, cosmic ray discoveries) – Retain. Blackett also did early paleomagnetism but Nobel cited cosmic rays; fine.
- 1949: Hideki Yukawa – (prediction of the pion, nuclear force theory) – Retain. Yukawa’s meson theory was the first significant theory from Japan recognized; well-deserved.
- 1950: Cecil Powell – (discovery of pion in cosmic rays, photographic emulsion technique) – Retain. Confirmed Yukawa’s particle experimentally.
- 1951: John Cockcroft & Ernest Walton – (first artificial nuclear disintegration by accelerator) – Retain.
- 1952: Felix Bloch & Edward Purcell – (NMR detection methods) – Retain.
- 1953: Frits Zernike – (phase-contrast microscope) – Retain (though it’s more of an invention impacting biology, still a physics technique).
- 1954: Max Born & Walther Bothe – (Born: foundational quantum theory; Bothe: coincidence method in particle detection) – Retain. We had awarded Born earlier in 1933; if so, Born might not get a second in 1954 (though he could). Perhaps in our timeline, in 1954 Born’s slot would be free. Bothe’s part we keep (he pioneered coincidence detection, important method).
- If Born is already laureate, we could replace him in 1954 with someone else omitted: Hendrik Kramers (quantum theory contributions, no Nobel), or Satyen Bose (but we did him), or Lev Landau (Landau actually got 1962 Nobel for superfluidity, but maybe earlier?). Landau did work across many fields by the 50s but his superfluid theory was 1941; recognition waited due to him nearly dying in 1962. Let's leave Landau to his actual year.
- 1955: Polykarp Kusch & Willis Lamb – (precision measurements: Lamb shift, magnetic moment of electron) – Retain. These experiments confirmed QED’s subtle predictions, we keep them.
- 1956: William Shockley, John Bardeen, Walter Brattain – (invention of the transistor) – Retain. This is applied physics but revolutionary, absolutely deserved.
- 1957: Tsung-Dao Lee & Chen Ning Yang – (parity violation theory) – Modify. We Add Chien-Shiung Wu. The parity violation was proven by Wu’s experiment, so she should share the 1957 Nobel. Thus 1957: T.-D. Lee, C.N. Yang, and C.S. Wu jointly. This corrects her omission.
- 1958: Pavel Cherenkov, Ilya Frank, Igor Tamm – (Cherenkov radiation explanation) – Retain.
- 1959: Emilio Segrè & Owen Chamberlain – (discovery of the antiproton) – Retain.
- 1960: Donald Glaser – (invention of the bubble chamber) – Retain (though some might debate it, the bubble chamber was quite impactful in particle discoveries).
Thus by 1960, our timeline has integrated the major mid-century oversights: Wu is recognized in 1957; Born earlier (so possibly not in 1954 but we replaced him with others as needed); Meitner in 1944; etc. The transistor guys remain, as that was fine.
3.6 1961–1970: The Space Age and Emergent Fields
The 1960s Nobels included cosmic discoveries and more particle/solid-state physics. Check for needed changes:
- 1961: Robert Hofstadter (nucleon structure via electron scattering) & Rudolf Mössbauer (Mössbauer effect) – Retain.
- 1962: Lev Landau – (theory of superfluidity, liquid helium) – Retain. Landau was a theoretical giant, deserved one; he got it.
- 1963: Eugene Wigner, Maria Goeppert Mayer, J. Hans Jensen – (Wigner: symmetries in physics; Mayer & Jensen: shell model of nucleus) – Retain. Wigner gets theory credit (some of which overlaps Noether’s concept, but his group theory in physics was huge), and Mayer (female laureate) & Jensen for nuclear shell model were right.
- 1964: Charles Townes, Aleksandr Prokhorov, Nikolay Basov – (maser/laser invention) – Retain.
- 1965: Richard Feynman, Julian Schwinger, Sin-Itiro Tomonaga – (quantum electrodynamics) – Retain. They formulated QED theory; absolutely.
- 1966: Alfred Kastler – (optical pumping) – Retain (though minor, we can keep).
- 1967: Hans Bethe – (stellar nucleosynthesis theory) – Retain. Bethe’s work on energy production in stars deserved it.
- 1968: Luis Alvarez – (bubble chamber work, particle discoveries) – Retain, albeit Alvarez is a bit controversial (he discovered many particle resonances, but so did many others).
- 1969: Murray Gell-Mann – (quark theory) – Retain. Fundamental contribution to particle theory.
- 1970: Hannes Alfvén & Louis Néel – (Alfvén: magnetohydrodynamics; Néel: magnetism in solids) – Retain.
Possible addition in the 60s:
- Jocelyn Bell Burnell’s pulsar discovery was 1967. The Nobel was given in 1974 to Hewish & Ryle. We will correct that in 1974.
- Perhaps 1965 could have also included Freeman Dyson for QED renormalization (Dyson never got Nobel, but he linked Schwinger & Feynman’s approaches). Usually only 3 are allowed, we already had 3. We can note Dyson but not necessarily add beyond 3.
- 1960s unknown unknowns: Some might argue for including someone like Claude Shannon, but he got a big prize in 1985 (Kyoto). We already recognized him earlier conceptually.
3.7 1971–1980: From the Standard Model to Astrophysics
The 1970s saw Nobels for the Standard Model pieces, new astrophysical phenomena, etc. Our adjustments:
- 1971: Dennis Gabor – (holography invention) – Retain.
- 1972: John Bardeen, Leon Cooper, Robert Schrieffer – (BCS theory of superconductivity) – Retain. (Bardeen’s second Nobel, as in reality.)
- 1973: Brian Josephson – (Josephson effect, superconducting tunneling) & Leo Esaki, Ivar Giaever – (tunneling in semiconductors and superconductors) – Retain. (Actually Josephson got half, Esaki/Giaever shared the other half).
- 1974: Antony Hewish & Martin Ryle – (pulsars & radio astronomy techniques) – Modify. Add Jocelyn Bell Burnell. In our timeline, 1974: Jocelyn Bell Burnell, Antony Hewish, Martin Ryle for pulsar discovery and radio astronomy. This corrects Bell’s exclusion.
- 1975: Aage Bohr, Ben Mottelson, James Rainwater – (nuclear structure collective model) – Retain.
- 1976: Burton Richter & Samuel Ting – (J/psi particle, charmonium discovery) – Retain.
- 1977: Philip Anderson, Nevill Mott, John van Vleck – (solid-state theory: disordered systems and magnetism) – Retain.
- 1978: Pyotr Kapitsa, Arno Penzias, Robert Wilson – (Kapitsa: superfluid helium experiments; Penzias/Wilson: discovery of cosmic microwave background) – Retain. CMB discovery was huge.
- 1979: Sheldon Glashow, Abdus Salam, Steven Weinberg – (electroweak unification theory) – Retain.
- 1980: James Cronin & Val Fitch – (CP violation in mesons) – Retain.
Notable omissions by 1980:
- Stephen Hawking: by 1980, Hawking had proposed Hawking radiation (1974). Some argued he deserved Nobel if it could be observed. Not yet observed, so he didn't get it. Our timeline could decide to award Hawking earlier on theoretical merit, but that would break the rule of needing experimental confirmation. Perhaps not give him yet (he never gets a Nobel in real history; we could consider giving him one in 1988 or something after indirect evidence of black holes evaporation? But none yet).
- Information/computing: by 1980 still no Nobel category. We already honored Turing/Shannon in our narrative as special cases.
- Teams: The 1978 CMB prize omitted theoretical discoverers (Gamow, Alpher, Herman who predicted CMB in 1940s, but Gamow was dead, others alive in 1978 but not recognized). One might say Ralph Alpher and Robert Herman deserved inclusion in 1978 for predicting CMB. In our timeline, since we allow multiple laureates, we could include them with Penzias and Wilson. But historically, Nobels rarely include theorists for something discovered long after. We can consider: 1978: Penzias, Wilson, and Alpher (say; Herman died 1997, Alpher 2007, they were alive in 1978). It’s a bit niche detail, but noteworthy because it addresses theory-experiment synergy. Let's mention it: in our Appendix, we list Alpher/Herman as co-laureates in 1978 for CMB prediction, to properly credit that insight.
- Quark model: Gell-Mann got 1969. George Zweig independently co-invented quark idea in 1964 but got no Nobel. Perhaps mention Zweig in passing but too late to add in 1969 because Gell-Mann alone is fine.
- We also note by 1980 only 2 women in physics had Nobels (Curie, Goeppert-Mayer; we added Wu, Meitner, Bell, Noether retroactively, making the gender balance slightly better in our timeline, but still far from equal).
3.8 1981–2000: Modern Physics and Missed Opportunities
For brevity, from the 1980s onward, Nobel choices are more recent. We highlight notable ones and check our list of under-appreciated:
- 1981: Nicolaas Bloembergen, Arthur Schawlow (laser spectroscopy); Kai Siegbahn (electron spectroscopy) – likely Retain.
- 1982: Kenneth Wilson – (critical phenomena, renormalization group) – Retain.
- 1983: Subrahmanyan Chandrasekhar & William Fowler – (Chandrasekhar: stellar structure theory; Fowler: nucleosynthesis) – Retain. (We had maybe given Chandra earlier, but if we didn't, he gets it now as in reality. If we did give him in 1943, then perhaps in 1983 we'd award someone else like Joseph Weber or others? But likely we didn't finalize Chandra earlier, so keep 1983 as is.)
- 1984: Carlo Rubbia & Simon van der Meer – (discovery of W/Z bosons at CERN) – Retain.
- However, note the W/Z discovery was a huge team effort (CERN’s UA1/UA2 collaborations). Rubbia was spokesman of UA1, van der Meer an engineer who made stochastic cooling for the accelerator. Our timeline might cite the CERN teams as part of 1984 recognition alongside these two individuals.
- 1985: Klaus von Klitzing – (quantum Hall effect) – Retain.
- 1986: Ernst Ruska (electron microscope); Heinrich Rohrer & Gerd Binnig (scanning tunneling microscope) – Retain.
- 1987: J. Georg Bednorz & K. Alex Müller – (high-Tc superconductivity) – Retain.
- 1988: Leon Lederman, Melvin Schwartz, Jack Steinberger – (muon neutrino beam method) – Retain.
- 1989: Norman Ramsey, Hans Dehmelt, Wolfgang Paul – (precision measurements: atomic clocks, ion traps) – Retain.
- 1990: Jerome Friedman, Henry Kendall, Richard Taylor – (deep inelastic scattering confirming quark structure) – Retain.
- 1991: Pierre-Gilles de Gennes – (soft matter physics) – Retain.
- 1992: Georges Charpak – (particle detectors) – Retain.
- 1993: Russell Hulse & Joseph Taylor Jr. – (binary pulsar, indirect gravitational wave evidence) – Retain. (This indirectly confirmed gravitational waves existence, which is interesting given Einstein wasn’t directly awarded for gravitational waves; they got it.)
- 1994: Bertram Brockhouse & Clifford Shull – (neutron scattering techniques) – Retain.
- 1995: Martin Perl (tau lepton discovery) & Frederick Reines (direct neutrino detection) – Retain.
- 1996: David Lee, Douglas Osheroff, Robert Richardson – (superfluid helium-3) – Retain.
- 1997: Steven Chu, Claude Cohen-Tannoudji, William Phillips – (laser cooling of atoms) – Retain.
- 1998: Robert Laughlin, Horst Störmer, Daniel Tsui – (fractional quantum Hall effect) – Retain.
- 1999: Gerard ‘t Hooft & Martinus Veltman – (renormalization in gauge theories) – Retain.
- 2000: Zhores Alferov, Herbert Kroemer, Jack Kilby – (semiconductor heterostructures; integrated circuit) – Retain.
During this period, some who never got Nobel:
- Stephen Hawking (still none; he died 2018). Perhaps we consider awarding Hawking around 1993 or 2016 when gravitational waves detected (though that’s more LIGO folks). Possibly 2016 we’ll mention.
- Joseph Weber (attempted gravitational wave detection, died 2000, not recognized; his early efforts are more historically interesting than Nobel-merit given he had false alarms).
- Andrei Sakharov (physicist who did work on controlled fusion and cosmic stuff, but he got a Peace Nobel for human rights).
- E.O. Lawrence was recognized (1939).
- Nikola Tesla we did in 1909.
- Other computing folks: We gave Turing/Shannon earlier notionally. Could consider awarding Tim Berners-Lee or Donald Knuth or others in computing? But beyond physics domain.
3.9 2001–2023: 21st Century – Completing the Picture
From 2001 onward, Nobel physics prizes have expanded into astrophysics and cosmology, quantum information, etc. We will mostly keep those, as they are current, but check for biases:
- Many of these recent prizes corrected previous omissions (e.g., acknowledging astrophysics, more women laureates like Donna Strickland 2018, Andrea Ghez 2020).
- Notable underrecognized now: Vera Rubin (dark matter evidence) – she died 2016, never got Nobel though many felt she should. Possibly mention awarding Vera Rubin posthumously or earlier for discovery of galactic rotation anomaly/dark matter evidence, maybe in 2020 along with Ghez and Penrose? But Ghez was for black holes, not dark matter.
- Possibly mention Bell’s Theorem test experiments – awarded in 2022 to Aspect, Clauser, Zeilinger. We wholeheartedly keep that, and note John Bell’s posthumous contribution.
- Actually in our timeline: John Bell could have been awarded in the 1980s if we allowed posthumous once experiments confirmed his theory (which they did by 1982 partially – Aspect’s experiments). John Bell died in 1990. We could have done 1982 or 1990: John Bell (theory of entanglement) as a special recognition, but now that 2022 Nobel indirectly honored him by giving his experimental testers the Nobel, we might list Bell as a co-laureate in 2022 posthumously (our rules allow) to explicitly credit the theory.
- 2013 Higgs: actual prize to Higgs & Englert. We already mentioned adding the other theorists conceptually and the experiments. Possibly note that in Appendix: 2013 – include Anderson, Kibble, Guralnik, Hagen, ’t Hooft, Brout (posthumously) along with Higgs/Englert. And mention LHC teams.
- 2017 gravitational waves: actual prize to Weiss, Barish, Thorne (LIGO). Should mention also credit to LIGO/Virgo collaborations in spirit.
- 2020: Penrose, Genzel, Ghez (black holes) – good.
- 2021: Syukuro Manabe, Klaus Hasselmann, Giorgio Parisi (climate modeling, complex systems) – good, and nice recognition of climate science.
- 2022: Aspect, Clauser, Zeilinger (quantum entanglement experiments) – good.
Given our timeline’s ethos:
- We would list Rubin as deserving – perhaps as co-laureate if say dark matter is ever recognized (no Nobel yet for dark matter discovery; maybe one day someone will, but the original evidence was hers).
- We include Bell (John) in 2022 implicitly (since we can break rules).
- We ensure team acknowledgments.
Unknown unknown current: Possibly mention that as of 2025, fields like quantum computing and quantum gravity still haven't fully gotten Nobels (quantum computing pioneers like Peter Shor, for example, or experimental quantum computer breakthroughs could in future). Or complex emergent phenomena, or biophysics intersection.
- Could say potential future: a Nobel for quantum computation (maybe to Shor and Deutsch and Grover, etc.) or quantum foundations (given 2022 was kind of that).
- Or exoplanets got a Nobel in 2019 (Mayor & Queloz).
- Perhaps a future Nobel for the discovery of extraterrestrial life if it happens, or for philosophical breakthroughs linking physics and consciousness (if any scientifically).
- Or mention that in a merit-only view, we might consider contributions like those of John von Neumann (theory of games, computing architecture, operator theory in QM) – he also never got Nobel since no category matched. We flagged some in earlier sections.
Now, after going through the timeline, it might be good to summarize:
Key Differences in the Alternative Timeline:
- Additional Laureates Added: Michael Faraday, Henri Poincaré, Emmy Noether, S.N. Bose, Chien-Shiung Wu, Jocelyn Bell Burnell, Lise Meitner, Nikola Tesla, etc., appear on the laureate list where they were absent historically.
- Controversial/less meritorious awards removed or shared: Philipp Lenard (1905) replaced by Boltzmann; Johannes Stark (1919) replaced by Einstein or others; perhaps Guillaume (1920) replaced by Bohr; etc.
- Posthumous awards introduced: Poincaré (died 1912, given 1912), Mendel (died 1884, retro 1865), Moseley (died 1915, maybe included 1921), Alpher (died later but we include him 1978).
- Multiple awards to same person where appropriate: e.g., Einstein (we gave him effectively two – one for relativity (1919) and originally he got one for photoelectric effect as in 1921 but we moved that; Fermi (1928 theory, 1938 experiment), Bardeen (1956 transistor, 1972 superconductivity in actual, we keep), maybe consider an Einstein multiple beyond what he got in reality.
- Team recognitions: acknowledging collaborations in narrative if not formal list (like LIGO, ATLAS/CMS).
- Interdisciplinary expansions: recognition of computing/information theory (Turing/Shannon), biology influence (Mendel, etc.), mathematics (Euler, etc.).
We might present a few comparative tables or bullet points but given the text length, perhaps summarizing in prose and highlighting some Appendices.
To conclude the chapter, we emphasize that the alternative timeline was an exercise revealing biases and structural limitations:
For example:
- Many more laureates in our timeline (since we allowed >3 and posthumous) – reflecting that science is a cumulative, cooperative enterprise.
- The presence of more women and international scientists – showing that contributions came from all quarters, even if historically they weren’t acknowledged due to bias or lack of Nobel categories.
- Some laureates in our timeline got recognized decades earlier than in reality (like Einstein or Born or Chandrasekhar), illustrating how social acceptance often lags scientific merit.
- The timeline also implicitly asks: if we had recognized all these earlier, would it have changed the course of science? Perhaps role models might have attracted more diversity earlier, etc.
We should mention these as reflections bridging to next chapters on biases and philosophical meaning.
Given the timeline complexity, we might not detail every year in the main text; perhaps highlight the major differences, which we did, and then say "see Appendix A for the full table."
Now, let's draft that in final.
Chapter 3: A Revised Nobel Prize Timeline (1901–2023)
We now integrate the insights of our merit-only analysis into a chronological alternative Nobel Prize timeline. In this timeline, each year’s Nobel Prize in Physics is reassigned based on true merit, free from original constraints. Where the original laureates were indeed the most deserving, we retain them; where omissions or biases occurred, we add or replace names. We also introduce retroactive honors for pre-1901 pioneers and allow multiple or posthumous awards as needed. Below, we summarize the key features of the revised timeline by era. (A detailed year-by-year list is provided in Appendix A, including both actual and revised laureates.)
3.1 Pre-1901 Retrospective Honors
Before the Nobel era, many foundational physicists never had a chance at such recognition. Our timeline accords them the equivalent of a Nobel in the year of or shortly after their defining contributions:
- 1687 (Retroactive): Isaac Newton – for “Principia Mathematica” (laws of motion, universal gravitation, calculus).
- 1750 (Retroactive): Leonhard Euler – for contributions to mechanics and mathematical physics.
- 1788 (Retroactive): Joseph Lagrange – for the Lagrangian formulation of mechanics.
- 1830s (Retroactive): Carl Friedrich Gauss – for work in electromagnetism and precision measurement.
- 1840 (Retroactive): Michael Faraday – for experimental discoveries in electromagnetism (e.g. induction).
- 1854 (Retroactive): Bernhard Riemann – for introducing differential geometry, enabling later relativity.
- 1865 (Retroactive): James Clerk Maxwell – for unifying electricity, magnetism, and light; and Gregor Mendel – for pioneering quantitative laws in biology (statistical genetics).
- 1890s (Retroactive): Ludwig Boltzmann – for statistical mechanics (we assign him a belated Nobel around 1905 when atomic theory was vindicated); J. Willard Gibbs – for foundational work in statistical mechanics and thermodynamics (assigned ~1902 posthumously); Heinrich Hertz – for discovery of radio waves (assigned ~1890); Nikola Tesla – for innovations in electric power and wireless technology (we will honor Tesla jointly in 1909 with Marconi).
Why these retrospectives? In real history, the early Nobel committees could only award living scientists, so Newton, Faraday, Maxwell, etc., never appear on Nobel rolls. Our merit-only narrative deliberately writes these giants back into the story. It shows that by 1901, the groundwork of classical physics was laid by many who never saw a Nobel Prize. This retrospective recognition underscores how heavily 20th-century physics built on these earlier contributions.
3.2 1901–1913: The Nobel Era Begins – Including the Overlooked
The first Nobel Prizes (1901–1913) in reality went to X-rays, radioactivity, and other major discoveries – all of which we keep. We make a few strategic additions:
- 1901: W. C. Röntgen (X-rays) – unchanged (the first Nobel prize remains with its deserving recipient).
- 1902: H. Lorentz & P. Zeeman (Zeeman effect, electron theory) – unchanged.
- 1903: Henri Becquerel, Marie Curie, Pierre Curie (radioactivity) – unchanged.
- 1904: Lord Rayleigh (argon gas discovery) – unchanged.
- 1905: Revised: We award Ludwig Boltzmann (statistical mechanics) in place of Philipp Lenard. Lenard’s work on cathode rays was important, but by 1905 Boltzmann’s once-controversial atomic theory had been empirically vindicated and deeply influenced the new quantum era. Boltzmann tragically died in 1906; honoring him in 1905 both recognizes a fundamental achievement and rectifies the Nobel committee’s historical preference for experimentalists over theorists. (Philipp Lenard is not awarded in our timeline, as his Nobel credit (cathode rays) was comparatively less paradigm-shifting, and his later anti-relativity stance tainted the merit of his recognition.)
- 1906: J. J. Thomson (electron discovery) – unchanged. Thomson’s identification of the electron remains a cornerstone and is retained (in our view, Thomson could have shared 1905 with Lenard in reality, but since we dropped Lenard, we allow Thomson’s own 1906 award to stand).
- 1907: A. A. Michelson (precision optical interferometry) – unchanged.
- 1908: G. Lippmann (color photography) – unchanged, though relatively minor, we keep this year’s original choice due to lack of a clearly superior alternative in 1908.
- 1909: Expanded: Guglielmo Marconi and Karl F. Braun (wireless telegraphy) joined by Nikola Tesla. The original 1909 prize credited Marconi and Braun for radio communication. We add Tesla as a third laureate, recognizing his equally crucial innovations in radio and electrical engineering. Tesla’s early radio experiments and AC power breakthroughs merit inclusion, correcting a famous historical omission – even the contemporary press speculated Tesla should have shared that Nobel. (Tesla had been considered for a Nobel but never received one; in our timeline, he does.)
- 1910: J. D. van der Waals (equation of state) – unchanged.
- 1911: W. Wien (Wien’s law of radiation) – unchanged.
- 1912: Added: Henri Poincaré – for “his contributions to mathematical physics, including the theory of relativity and the three-body problem.” In reality, no Nobel was awarded in 1912. We seize that opportunity to honor Poincaré, who died that year after being nominated a record 51 times. Poincaré’s sweeping contributions – from nearly discovering special relativity to founding topology and chaos theory – were passed over by the committee because they couldn’t pinpoint one discovery and due to lingering doubts about relativity. Merit-only analysis dictates that such a polymath be recognized. We posthumously give Poincaré the Nobel in 1912, symbolically acknowledging that he “truly deserved recognition” where history hesitated.
- 1913: H. Kamerlingh Onnes (helium liquefaction, superconductivity) – unchanged.
Outcome by 1913: All actual laureates up to 1913 remain except Lenard and the un-awarded Stark (1919) case we handle later. In addition, Boltzmann, Tesla, and Poincaré have entered the Nobel roster. This early revision already addresses a theory-vs-experiment bias (Boltzmann, Poincaré) and a geopolitical bias (Tesla, an outsider to the European elite). Notably, Marie Curie (1903) remains the only female laureate so far; but others are on the horizon in our timeline (Noether, Meitner, etc.).
3.3 1914–1929: Relativity and Quantum Revolution – Accelerated Recognition
This period covers World War I and the roaring 1920s – when Einstein’s relativity was confirmed and quantum mechanics was born. Our timeline speeds up recognition of groundbreaking theoretical work and ensures wartime biases are mitigated:
- 1914: Max von Laue (X-ray diffraction) – unchanged.
- 1915: W. L. Bragg & W. H. Bragg (X-ray crystallography) – unchanged.
- 1916: (Prize not awarded due to WWI) – In reality, none. In our timeline we also withhold in 1916 (no major alternative award is inserted; relativity was not yet empirically confirmed).
- 1917: (No award, WWI) – unchanged.
- 1918: Max Planck (quantum theory) and Emmy Noether (symmetry and conservation). We expand 1918’s prize to a dual award. Planck’s quantum hypothesis (origin of quantum physics) certainly merits the Nobel he received. We add Emmy Noether: her 1918 theorem linking symmetries to conservation laws is perhaps the single most profound insight in physics that a Nobel was never given for. In our narrative, Noether’s theorem – “every symmetry corresponds to a conserved quantity” – is fully appreciated as fundamental truth. Despite being a woman in a male-dominated era, she is now recognized in 1918 alongside Planck. This corrects both a gender bias and the undervaluing of mathematical physics: “Mathematical foundations are as important as experimental discoveries”, and Noether provided exactly that foundation. (Historical note: Noether was overlooked partly due to sexism and her work’s abstract nature. Our timeline proudly breaks those barriers.)
- 1919: Revised: Albert Einstein – for “the theory of general relativity, confirmed by the 1919 eclipse observations, and his contributions to theoretical physics.” In actuality, 1919’s Nobel went to Johannes Stark (for splitting spectral lines in electric fields), a choice later criticized as politically motivated and scientifically less significant. We replace Stark with Einstein, effectively awarding Einstein a Nobel several years earlier than he actually received one. The dramatic 1919 measurement of starlight bending by the Sun proved Einstein’s general relativity correct – a result that captivated the world. In our timeline, the Nobel Committee acts without delay to honor Einstein in 1919 for relativity, rather than waiting until 1922 (and then awkwardly citing only the photoelectric effect). This timely recognition asserts that empirical proof of a new theory of gravity is as deserving as any discovery. (Stark’s Nobel is dropped; his case is a clear example where merit was entangled with nationalist bias, which we eliminate.)
- 1920: Revised: Niels Bohr – for “the quantum model of the atom.” The 1920 prize in reality went to Charles E. Guillaume for an alloy (Invar) – a useful but not world-changing discovery. We supplant Guillaume with Bohr, whose 1913 atomic theory was the cornerstone of early quantum physics. Historically, Bohr was awarded (in 1922) after a delay; our timeline accelerates credit to Bohr in 1920, reflecting the immediate and fundamental impact of his atomic model on physics and chemistry. Guillaume’s recognition, which likely reflected geographic/institutional considerations of the committee, is dropped in favor of Bohr’s truly paradigm-shifting work.
- 1921: Revised: Ernest Rutherford – for “discoveries in nuclear physics, including the atomic nucleus and proton.” In actuality, 1921 had no physics Nobel awarded (and the 1921 prize was later given to Einstein in 1922). Having already awarded Einstein, we use 1921 to honor Rutherford. Rutherford, though he won the 1908 Chemistry Nobel for early radioactivity work, never received a Physics Nobel. By 1921, his achievements included discovering the atomic nucleus (1909) and the proton (1919), essentially founding nuclear physics. We grant Rutherford a Physics Nobel to underscore his pivotal role in understanding atomic structure. Additionally, we posthumously include Henry Moseley as a co-laureate in 1921 for establishing atomic number order (Moseley’s 1913 X-ray spectra proved that elements are defined by proton number). Moseley died in WWI (1915) at age 27, widely mourned as a scientific genius cut down. In our timeline, his contribution – essential to the periodic table and nuclear theory – is acknowledged alongside Rutherford. This joint award in 1921 rectifies a wartime tragedy (Moseley’s lack of Nobel due to early death) and completes the picture of the atom’s internal architecture.
- 1922: Arthur Compton (Compton effect) and Otto Stern & Walther Gerlach (spin quantization experiment). Historically, 1922’s Nobel went to Bohr (which we moved to 1920). We repurpose 1922 to recognize key quantum experiments: Compton’s 1923 discovery of the particle nature of X-ray photons, and the 1922 Stern–Gerlach experiment demonstrating space quantization of atomic spin. In reality, Compton got the 1927 Nobel and Stern shared a delayed 1943 Nobel (Gerlach received none). We award them earlier, in the same year as their findings, to reflect immediate merit. Thus, 1922 is shared by Arthur Compton, Otto Stern, and Walther Gerlach – highlighting that the quantum revolution was driven by both theoretical insight and brilliant experimentation in tandem.
- 1923: Robert Millikan (electron charge & photoelectric effect measurement) – unchanged.
- 1924: Manne Siegbahn (X-ray spectroscopy) – unchanged.
- 1925: James Franck & Gustav Hertz (quantum inelastic collisions in atoms) – unchanged.
- 1926: Jean Perrin (confirmation of Brownian motion, atomic reality) – unchanged.
- 1927: C.T.R. Wilson (cloud chamber) & Arthur Compton – slight change: In real 1927, Compton and Wilson shared the prize. In our timeline, Compton was already honored in 1922, so 1927 can fully recognize Charles T. R. Wilson (for the cloud chamber) and possibly add Patrick Blackett (who used the cloud chamber to discover particle interactions, and later got a Nobel in 1948 for cosmic rays). However, to avoid confusion, we can leave 1927 solely to Wilson, acknowledging the cloud chamber’s importance for particle physics.
- 1928: Expanded: Satyendra Nath Bose and Enrico Fermi – for “statistical mechanics of quantum systems (Bose–Einstein and Fermi–Dirac statistics)”. Originally 1928’s Nobel went to Owen Richardson (thermionic emission), a relatively modest contribution. We instead recognize that by the late 1920s, quantum theory had branched into quantum statistics, thanks to Bose and Fermi. S.N. Bose’s 1924 work and Enrico Fermi’s 1926 work introduced two classes of particles (bosons and fermions) and explained quantum behavior of gases. These ideas later led to predictions like the Bose–Einstein condensate (confirmed much later) and underlie the entire edifice of condensed matter and nuclear physics. Historically, Bose never won a Nobel, and Fermi’s Nobel (1938) was for experimental work. By awarding them jointly in 1928, we acknowledge that the conceptual framework they provided was as significant as any single experiment. It also brings an Indian scientist (Bose) into the laureate ranks early, correcting geographic imbalance.
- 1929: Louis de Broglie (wave nature of electrons) – unchanged.
By 1929, our alternate timeline has dramatically enriched the Nobel roster. Einstein appears (1919) for relativity, rather than being delayed and then cited for a lesser discovery. Bohr appears promptly (1920). Noether and Bose, otherwise absent from Nobel history, are now included. We have also honored Rutherford and Moseley for unraveling atomic structure, and given parity to key experimental tests (Stern-Gerlach, Compton effect) right when they occurred, rather than much later. The result is a 1920s Nobel landscape that more accurately reflects the true revolution sweeping physics: relativity and quantum theory are front and center, and the global and diverse cast of contributors – including a woman (Noether) and scientists from India (Bose) and New Zealand (Rutherford) – are recognized on merit.
3.4 1930–1945: New Particles, New Insights – Fulfilling Missed Nobels
The 1930s Nobels in our timeline continue to match many actual awards for the blossoming fields of quantum mechanics, particle physics, and nuclear physics, but with pivotal corrections for those overlooked or undervalued:
- 1930: C. V. Raman (Raman effect) – unchanged. Raman’s light-scattering discovery, made in India, is retained as in reality.
- 1931: (No award) – unchanged.
- 1932: Werner Heisenberg – unchanged (quantum mechanics and the Uncertainty Principle).
- 1933: Erwin Schrödinger, Paul Dirac, and Max Born – (foundations of quantum mechanics). In reality, Schrödinger and Dirac shared 1933, while Max Born was belatedly honored in 1954. We include Born in 1933 as a third laureate for his fundamental contributions (matrix mechanics, and later the statistical interpretation of the wavefunction). Born’s omission in the 1930s was a quirk – arguably due to politics (he fled Nazi Germany) and timing. Our timeline fixes that: Schrödinger, Dirac, and Born jointly represent the complete triumph of quantum theory.
- 1934: (No award) – We assign Enrico Fermi here for his 1934 experiments bombarding nuclei with neutrons, which created new radioactive isotopes (the first artificial elements). Historically, Fermi’s group unknowingly achieved nuclear fission precursors and transuranic elements. We award 1934: Enrico Fermi (his first Nobel) to mark these pioneering neutron experiments which ushered in the nuclear era. (Fermi will appear again for later work – one of the few double Nobelists in our timeline, reflecting his outsize merit.)
- 1935: James Chadwick – unchanged (discovery of the neutron).
- 1936: Victor Hess & Carl Anderson – unchanged (Hess: cosmic rays; Anderson: discovery of the positron).
- 1937: Clinton Davisson & George Thomson – unchanged (electron diffraction confirming matter waves).
- 1938: Enrico Fermi – unchanged (for slow neutron-induced radioactivity). This is Fermi’s second Nobel in our timeline – by 1938, his experimental work splitting atomic nuclei earned him the prize (as in reality). Thus Fermi is now a two-time laureate (1928 for theory with Bose, 1938 for experiment) – a scenario the actual Nobel rules wouldn’t allow, but we permit because merit warrants it. (Only John Bardeen in reality won twice in physics; in our version, Fermi joins that elite club for his singular contributions across domains.)
- 1939: Ernest Lawrence – unchanged (invention of the cyclotron particle accelerator).
- 1940–1942: (No awards, WWII) – unchanged.
- 1943: Otto Stern – Stern was already honored in 1922 in our timeline, so 1943 is free. We use 1943 to posthumously acknowledge Subrahmanyan Chandrasekhar for his 1930s work on stellar structure (Chandrasekhar limit for white dwarfs). In reality, Chandrasekhar’s work was initially belittled (due to Eddington’s resistance) and he waited until 1983 for a Nobel. In our merit-based view, the importance of his result – predicting black hole formation when stars exceed a mass limit – was evident by the 1930s in hindsight. We award Chandrasekhar in 1943 to recognize theoretical astrophysics as part of physics’ advancement (and again broaden geographic representation, as an Indian-origin laureate). This is admittedly a speculative correction, relying on historical hindsight of how crucial Chandrasekhar’s limit became. It serves to show our framework’s willingness to correct delayed appreciation of merit. (Stern’s 1943 Nobel for molecular beams was deserved, but he was already recognized earlier in our timeline.)
- 1944: Isidor Rabi – unchanged (nuclear magnetic resonance). Additionally, we award Lise Meitner in 1944. The discovery of nuclear fission (splitting the uranium atom) rocked physics in 1938. Otto Hahn received the 1944 Chemistry Nobel for it, but Lise Meitner, who co-discovered and explained fission, was excluded. Our timeline gives Lise Meitner a Nobel Prize in Physics (1944), citing her “critical contribution to discovering and understanding nuclear fission.” This corrects one of the most glaring Nobel oversights attributed to gender bias and wartime politics. Meitner shares this 1944 Physics honor in our list (perhaps alongside her nephew Otto Frisch, who worked with her). Hahn can keep the Chemistry Nobel for the experimental part, but Meitner now rightfully appears in the Physics Nobel record as the “forgotten mother of fission”.
- 1945: Wolfgang Pauli – unchanged (exclusion principle in quantum theory).
By 1945, our Nobel timeline has a notably richer cast. It includes Meitner (female, Jewish-Austrian exile) as a laureate when in reality she was ignored, and Chandrasekhar decades before his actual prize. Einstein, Bohr, Born, Fermi (twice), Noether, Bose, Poincaré, Tesla, Boltzmann, Rutherford, Moseley, Stern-Gerlach, Wu, Bell – all these names appear by mid-century, whereas in real history many were absent or came much later. The wartime and interwar biases (which favored certain nationalities or personalities) are smoothed out: we did not hesitate to honor contributions from German-Jewish refugees (Born, Meitner) or from scientists in the Global South (Raman, Bose, Chandrasekhar). Also, by 1945 our timeline has four women in Physics Nobels (Curie, Noether, Meitner, Wu (coming in 1957), and soon Chien-Shiung Wu and Jocelyn Bell). This is still modest, but far above the single woman (Curie) that reality had by 1945, highlighting that many women did contribute fundamentally but were left out originally due to bias.
3.5 1946–1970: Big Science, Big Teams – Sharing the Spotlight
In the post-WWII era, physics became “big science”: huge collaborations, expensive machines, and an explosion of subfields (solid-state, particle, space physics). The Nobel awards of this era in our timeline largely mirror the actual ones – the Nobel committees in these decades did a reasonable job selecting important advances – but we modify a few to acknowledge team efforts and previously overlooked individuals:
- 1946–1949: Prizes for experimental and solid-state physics are unchanged: e.g., P. Bridgman (high-pressure physics, 1946), E. Appleton (ionospheric physics, 1947), P. Blackett (cosmic rays, 1948), H. Yukawa (meson theory, 1949).
- 1950: Cecil Frank Powell (discovering pions in cosmic rays) – unchanged.
- 1951: Cockcroft & Walton (first artificial nuclear disintegration) – unchanged.
- 1952: Felix Bloch & Edward Purcell (NMR methods) – unchanged.
- 1953: Frits Zernike (phase-contrast microscopy) – unchanged.
- 1954: Max Born & Walther Bothe – Revised since we awarded Born in 1933. We let Walther Bothe (coincidence method in particle detection) keep the prize, and instead of Born, we add Chien-Shiung Wu here as an anticipatory move. However, Wu’s major contribution (parity violation experiment) happens in 1956, so perhaps it’s premature. Alternatively, since Born was pre-awarded, we could honor Hendrik Kramers or Arnold Sommerfeld in 1954. Sommerfeld mentored many Nobelists and advanced atomic physics, but he was never awarded; Kramers (Dutch theorist) contributed to quantum theory and dispersion relations. This is a subtle gap: a 1954 slot opens. We might choose Arnold Sommerfeld for his lifetime of contributions to quantum theory (this would posthumously acknowledge a giant teacher/researcher who never got a Nobel). Sommerfeld died in 1951; giving him a memorial Nobel in 1954 alongside Bothe would salute the role of mentorship and theory in physics. We’ll assume 1954: Walther Bothe & Arnold Sommerfeld (posth.).
- 1955: Willis Lamb & Polykarp Kusch (precision QED measurements) – unchanged.
- 1956: Shockley, Bardeen, Brattain (transistor) – unchanged.
- 1957: T. D. Lee & C. N. Yang – expanded: + Chien-Shiung Wu. The 1957 prize in reality honored Lee and Yang for theoretically predicting parity violation. We add Wu, whose 1956 experiment proved their prediction by showing that nature distinguishes left from right. Historically, Wu was shockingly omitted (likely due to being a woman and an experimentalist). In our timeline, 1957: Lee, Yang, and Wu jointly receive the Nobel Prize. This reinforces a theme: theorist and experimentalist should share credit for a discovery of such magnitude, and it corrects the gender bias that left Wu out. (This also marks the fifth female physics laureate in our list by 1957 – Marie Curie, Noether, Meitner, Wu, and perhaps Goeppert-Mayer in 1963 – whereas historically only two women had won by then.)
- 1958–1960: Prizes for Cherenkov radiation (1958), the antiproton discovery (1959), and the laser & maser principles (1960) – unchanged.
- 1961: Robert Hofstadter (nucleon structure) & Rudolf Mössbauer (gamma resonance) – unchanged.
- 1962: Lev Landau (theory of superfluids) – unchanged.
- 1963: E. Wigner, Maria Goeppert Mayer, J. Jensen (nuclear shell model and symmetry theory) – unchanged.
- 1964: Charles Townes, Nicolay Basov, Aleksandr Prokhorov (maser/laser) – unchanged.
- 1965: Richard Feynman, Julian Schwinger, Sin-Itiro Tomonaga (quantum electrodynamics) – unchanged (we already accounted for Dyson’s role via inclusion of Freeman Dyson as an honorable mention, though not a laureate).
- 1966: Alfred Kastler (optical pumping) – unchanged.
- 1967: Hans Bethe (stellar nucleosynthesis) – unchanged.
- 1968: Luis Alvarez (particle detectors, resonance discoveries) – unchanged, though we note that many colleagues contributed to particle discoveries; Alvarez still merits recognition for innovation in experimental techniques.
- 1969: Murray Gell-Mann (quark model) – unchanged (we recognize George Zweig’s independent co-discovery of quarks in passing, but Nobel can only go to three and Gell-Mann was clearly deserving).
- 1970: Hannes Alfvén (magnetohydrodynamics) & Louis Néel (antiferromagnetism) – unchanged.
During this period, team science became prominent. Our timeline doesn’t change the laureates but explicitly acknowledges collaborations in narratives. For example:
- 1957: Along with Lee, Yang, Wu, we implicitly credit the many personnel involved in the parity experiments (Wu’s low-temperature team at NBS) – a nod to the collaborative nature of modern physics.
- 1960s: Discovery of the quark (Gell-Mann 1969) relied on deep inelastic scattering experiments (awarded in 1990 to Friedman, Kendall, Taylor). We maintain the original schedule but highlight that many experimental teams supported these breakthroughs. Our alternative history would not shy from mentioning, in Nobel citations or press releases, the contributions of groups like the MIT-SLAC collaboration for quark evidence, even if the prize goes to an individual like Gell-Mann.
- Big science facilities: In 1968 Alvarez’s work used large bubble chambers and teams; in our narrative we ensure those team efforts are praised, though the Nobel remains with Alvarez.
By 1970, essentially all major theoretical structures of physics (relativity, quantum mechanics, the quark model, etc.) and major experimental discoveries (particles, lasers, superconductivity) have been recognized in our timeline in a timely manner. The standard model of particle physics is nearly complete (Glashow, Salam, Weinberg come in 1979, which we’ll keep).
We have also systematically corrected earlier blind spots: Wu is in; Meitner is in; Bell (coming 1974) will be in; Noether, Bose, Chandrasekhar are in; etc. Moreover, the Nobel roster by 1970 is far more diverse – multiple laureates from Asia (Raman, Bose, Chandrasekhar, Yang, Lee, Yukawa, Tomonaga, etc.), Eastern Europe (Čerenkov, Ginzburg later, etc.), and an increased female presence. Merit, after all, knows no borders or gender – and now our Nobel timeline reflects that truth.
3.6 1971–1990: The Standard Model, Astrophysics, and Computing – Setting Things Right
From the 1970s through 1980s, Nobel prizes recognized the completion of the Standard Model of particle physics and opened to astrophysics and cosmology. We adopt most of these awards unchanged, as they align well with merit, but make key inclusions:
- 1971–1975: Prizes to Dennis Gabor (holography, 1971), Bardeen-Cooper-Schrieffer (superconductivity theory, 1972), Leo Esaki, Ivar Giaever, Brian Josephson (quantum tunneling, 1973), and Bohr/Mottelson/Rainwater (nuclear structure, 1975) – all unchanged.
- 1974: Antony Hewish & Martin Ryle – expanded: + Jocelyn Bell Burnell. The 1974 Nobel credited Ryle and Hewish for the discovery of pulsars, but infamously left out Jocelyn Bell, who as a graduate student actually discovered the pulsar signal. This omission is widely regarded as one of the greatest Nobel slights, attributed to Bell’s junior status and perhaps gender bias. In our timeline, 1974: Hewish, Ryle, and Bell Burnell share the Nobel Prize for pulsars and radio astrophysics. This sets the record straight: Bell’s pivotal role is finally recognized alongside her supervisor and the radio astronomy innovator. As Bell’s case shows, merit-based corrections sometimes mean crediting the true discoverer regardless of title or hierarchy – a practice our timeline embraces to uphold truth. (Bell’s inclusion also further elevates the representation of women in Nobel history.)
- 1976: Burton Richter & Samuel Ting (discovery of J/ψ particle, confirming the charm quark) – unchanged.
- 1977: Philip Anderson, Nevill Mott, John van Vleck (theory of condensed matter, electron localization and magnetism) – unchanged.
- 1978: Pyotr Kapitsa (superfluidity experiments) & Arno Penzias, Robert Wilson (cosmic microwave background discovery) – modified: We add theoretical cosmologists Ralph Alpher and Robert Herman to the 1978 prize. Penzias and Wilson stumbled on the CMB in 1964, but Alpher and Herman had in 1948 predicted such radiation as a remnant of the Big Bang. In reality they were never recognized by a Nobel. In our timeline, we allow a fourth name (breaking the “rule of three”) to honor Alpher (Herman died in 1997, but could also be acknowledged posthumously). Thus, 1978: Penzias, Wilson, Alpher (and Herman) collectively for the empirical discovery and prior prediction of the CMB. This emphasizes that discovering truth sometimes involves both predicting it and finding it – a synergy our merit awards can reflect. (The Nobel committees have rarely done this joint theorist-experimentalist awarding after a long gap, but we choose to, as principle.)
- 1979: Steven Weinberg, Abdus Salam, Sheldon Glashow (electroweak unification theory) – unchanged.
- 1980: James Cronin & Val Fitch (CP violation in mesons) – unchanged.
- 1981–1983: Prizes to Kai Siegbahn, Nicolaas Bloembergen, Arthur Schawlow (laser spectroscopy, 1981); Kenneth Wilson (critical phenomena, 1982); Subrahmanyan Chandrasekhar & William Fowler (stellar evolution, 1983) – unchanged. (Chandrasekhar we already honored in 1943, but it’s fine that he appears again here in reality. If we consider our timeline strictly, Chandra wouldn’t get a “second” Nobel in 1983 since he’s already recognized. We might instead use 1983 to honor, say, Yaakov Zeldovich or Edward Lorenz or others in astrophysics/chaos who were overlooked. However, we can also accept that sometimes people might earn two Nobels – Chandrasekhar’s case is borderline since it’s the same work being recognized late. We’ll assume our timeline noted his 1943 award and thus in 1983 the committee might instead honor someone like Ya. Zeldovich (a Soviet physicist who co-predicted elements of cosmology and black hole theory) alongside Fowler. This is speculative – we leave it open as an example of flexibility.)
- 1984: Carlo Rubbia & Simon van der Meer (W and Z boson discovery at CERN) – unchanged. But we explicitly note that the 1984 Nobel press release in our timeline would laud the entire CERN UA1/UA2 teams that found those bosons. In merit terms, the discovery of the W/Z was a triumph of hundreds of scientists; we can’t list them all as laureates, but we break the convention by at least acknowledging the collaboration by name. Our timeline sets a precedent that big-team discoveries come with official recognition of the team effort (even if the gold medal is given to spokespeople like Rubbia).
- 1985–1986: Klaus von Klitzing (quantum Hall effect, 1985); Ernst Ruska (electron microscope), Gerd Binnig & Heinrich Rohrer (scanning tunneling microscope) – unchanged.
- 1987: J. Georg Bednorz & K. Alex Müller (high-Tc superconductivity) – unchanged.
- 1988: Leon Lederman, Melvin Schwartz, Jack Steinberger (muon neutrino beam, 1988) – unchanged.
- 1989: Norman Ramsey, Hans Dehmelt, Wolfgang Paul (atomic clock and ion trap techniques) – unchanged.
- 1990: Jerome Friedman, Henry Kendall, Richard Taylor (deep inelastic scattering confirming quark structure) – unchanged. We highlight that this 1990 award is essentially the experimental complement to the 1969 Gell-Mann award – in our timeline we explicitly connect the two, noting Gell-Mann’s quark theory was validated by Friedman, Kendall, Taylor’s work. The combination shows how merit was distributed over time; our commentary makes that synergy clear, which Nobel history often leaves implicit.
- 1991–1993: Pierre-Gilles de Gennes (soft matter physics, 1991); Georges Charpak (particle detectors, 1992); Russell Hulse & Joseph Taylor Jr. (binary pulsar – indirect gravitational waves, 1993) – unchanged.
- 1994–1995: Bertram Brockhouse & Clifford Shull (neutron scattering, 1994); Martin Perl & Frederick Reines (tau lepton discovery and neutrino detection, 1995) – unchanged.
- 1996–1997: David Lee, Douglas Osheroff, Robert Richardson (superfluid helium-3, 1996); Steven Chu, Claude Cohen-Tannoudji, William Phillips (laser cooling, 1997) – unchanged.
- 1998: Robert Laughlin, Horst Störmer, Daniel Tsui (fractional quantum Hall effect) – unchanged.
- 1999: Gerard ‘t Hooft & Martinus Veltman (electroweak interactions renormalization) – unchanged.
- 2000: Zhores Alferov, Herbert Kroemer, Jack Kilby (semiconductor heterostructures and integrated circuits) – unchanged.
During the 1980s, a notable omission was Stephen Hawking. Hawking’s theoretical prediction of black hole evaporation (Hawking radiation) in 1974 was groundbreaking, but could not be experimentally confirmed (and still hasn’t directly). Nobel criteria demand observations – thus Hawking never won. In our merit-only philosophy, one could argue Hawking’s contribution is so deep that it merited recognition regardless of experimental proof, as an insight into quantum gravity and thermodynamics. One could imagine a special Nobel around the late 1980s recognizing “Stephen Hawking, for theoretical discoveries at the nexus of gravitation and quantum mechanics (black hole thermodynamics)”. This would be a bold break – essentially rewarding a prediction before confirmation. Historically, the Nobel committee never does this. Our timeline so far has also required actual discoveries or at least widely accepted theories. Hawking occupies a gray zone: widely accepted (by theoretical consensus) but not empirically verified. We leave Hawking unawarded up to 2000, noting this as a remaining limitation of the Nobel process – even in our improved timeline, we hesitated to give a prize without experimental support. We will revisit this in Chapter 5’s philosophical discussion on “unknown unknowns” and the evolving criteria of truth. (We might ultimately decide to give Hawking a posthumous honorary Nobel in the appendix list, to illustrate ideal merit recognition for unproveable-but-likely truths.)
Another person in this era: Claude Shannon (the father of information theory) – we already recognized him earlier conceptually (with a hypothetical mid-century prize alongside Turing). By 2000, computing and information science had revolutionized society, yet still no Nobel category covers them. Our timeline might show a footnote that in 1985 Shannon received the Kyoto Prize (called “Japan’s Nobel”), implicitly acknowledging the Nobel gap. In a fully merit-merit world, perhaps a one-off Nobel in Physics could have been given to Shannon or Turing, arguing that information is physical. We’ve effectively done that by mentioning a joint Turing-Shannon award in the mid-20th century section. By 2000, the influence of their work is undeniable (the entire digital age). We count them as honorary laureates in our account, even if not in the official Nobel categories.
3.7 2001–2023: New Frontiers – Completing the Merit Timeline
In the 21st century, Nobel Prizes in Physics have further diversified: recognizing accelerating universe (dark energy), exoplanets, quantum information, and more. Our timeline here largely mirrors reality, which has recently started addressing some past blind spots:
- 2001–2005: Prizes for Bose–Einstein condensates (2001), astrophysical discoveries (neutrino oscillations in 2002, precision cosmology in 2006), theory of broken symmetry (2008), etc., are unchanged, as the Nobel Committee by and large picked worthy achievements. (Notably, 2003: Vitaly Ginzburg, Alexei Abrikosov, Anthony Leggett for superconductivity/superfluidity theory – Ginzburg was a name long deserving; 2004: Gross, Politzer, Wilczek for quark confinement (QCD) – good picks.)
- 2006: John Mather & George Smoot (mapping the CMB) – unchanged (this builds on 1978’s CMB discovery; we might note that our timeline had already credited Alpher in 1978 for the concept).
- 2007: Peter Grünberg & Albert Fert (giant magnetoresistance, enabling modern hard drives) – unchanged.
- 2008: Yoichiro Nambu, Makoto Kobayashi, Toshihide Maskawa (spontaneous symmetry breaking & quark mixing) – unchanged.
- 2009: Charles Kao (fiber optics), Willard Boyle & George Smith (CCD sensors) – unchanged.
- 2010: Andre Geim & Konstantin Novoselov (graphene) – unchanged.
- 2011: Saul Perlmutter, Brian Schmidt, Adam Riess (dark energy, accelerating universe) – unchanged.
- 2012: Serge Haroche & David Wineland (quantum optics, controlling quantum systems) – unchanged.
- 2013: François Englert & Peter Higgs – expanded: + Robert Brout, Gerald Guralnik, C. R. Hagen, Tom Kibble, Philip Anderson, and ATLAS/CMS collaborations. The 2013 prize went to Higgs and Englert for the theory of the Higgs boson. But six people (including Higgs and Englert) had independently contributed to that theory in 1964, and only Nobel’s three-person rule prevented the others from sharing. Additionally, the actual particle’s discovery in 2012 was by thousands at CERN (ATLAS & CMS). Our timeline’s ethos dictates inclusivity: we list Higgs, Englert, Brout (posthumously), Guralnik, Hagen, Kibble, and Anderson as collectively having deserved the theoretical credit, and we explicitly acknowledge the experimental collaborations at CERN in the Nobel citation. While logistically one can’t have 1000 Nobel medals, in spirit we break the barrier here: the Higgs mechanism is recognized as a convergence of multiple minds and machines. (Brout died in 2011; we would waive the posthumous restriction to include him, as we did for others.)
- 2014: Isamu Akasaki, Hiroshi Amano, Shuji Nakamura (blue LEDs) – unchanged.
- 2015: Takaaki Kajita & Arthur McDonald (neutrino oscillations) – unchanged.
- 2016: David Thouless, Duncan Haldane, J. Michael Kosterlitz (topological phases of matter) – unchanged.
- 2017: Rainer Weiss, Kip Thorne, Barry Barish (gravitational wave detection) – unchanged, but we emphasize that this award represents the LIGO Scientific Collaboration as a whole. In our timeline, the Nobel Committee, for the first time, formally mentions the entire LIGO/Virgo team in the award citation, even if the medal goes to the three spokespersons. This sets a precedent aligning with our merit ideals: major collaborations get recognized as entities. (Modern Nobel announcements have started to nod to teams; we amplify that.)
- 2018: Arthur Ashkin, Gérard Mourou, Donna Strickland (laser physics) – unchanged. We note Donna Strickland is (in reality and our timeline) the third woman to win a Physics Nobel (after Curie and Goeppert-Mayer). In our alternate timeline count, she is actually the seventh female Physics laureate (Curie, Noether, Meitner, Wu, Goeppert-Mayer, Bell, Strickland – plus Jocelyn Bell Burnell now recognized).
- 2019: James Peebles (cosmology theory), Michel Mayor & Didier Queloz (exoplanets) – unchanged. We might append Vera Rubin here as a posthumous co-laureate with Peebles, acknowledging her pioneering work on galaxy rotation and dark matter in the 1970s. Rubin never got a Nobel (she died in 2016, ironically the year before dark matter’s indirect evidence was honored via other means in 2019’s Chemistry Nobel to cosmologist Jim Peebles). Our timeline could bend rules to honor Vera Rubin alongside Peebles in 2019, stating: “for contributions to our understanding of the universe’s structure (dark matter and cosmology).” This would right a wrong for one of the most famous female astronomers. Given Peebles already got the full half of the 2019 prize, in our alternate scenario we’d give one quarter each to Peebles and Rubin. This again demonstrates our willingness to correct the record (Rubin’s exclusion was widely criticized).
- 2020: Roger Penrose, Reinhard Genzel, Andrea Ghez (black holes) – unchanged. We note Penrose’s theoretical work on black hole formation was long unrecognized; the Nobel committee did well to finally honor it (Penrose might arguably have deserved an earlier Nobel for his contributions to general relativity and twistor theory, but 2020 was apt with empirical confirmation of black holes). Andrea Ghez is now another female laureate in our timeline (adding to our enriched list).
- 2021: Syukuro Manabe & Klaus Hasselmann (climate modeling); Giorgio Parisi (complex systems) – unchanged. This prize is notable for expanding into climate science and recognizing long-term impact on understanding complex physical systems – very much in line with our merit focus on long-term, foundational contributions. We applaud that and keep it.
- 2022: Alain Aspect, John Clauser, Anton Zeilinger (quantum entanglement experiments) – unchanged, except we add John S. Bell (posthumously) as an honorary fourth laureate. The 2022 prize was essentially “the Bell’s theorem prize,” celebrating experiments that proved Bell’s 1964 theoretical inequalities and thus quantum nonlocality. Bell himself died in 1990, never having been recognized by a Nobel. Our timeline, removing the posthumous restriction, lists John Bell alongside Aspect, Clauser, Zeilinger as the theoretical originator whose insight made those experiments meaningful. This provides closure to a long-running omission: Bell’s name is now where it scientifically belongs – on the same pedestal as the experimentalists who confirmed his genius idea. It also signals that our merit-only policy values fundamental theory on par with experiment, even if fate didn’t allow the theorist to live to see vindication.
- 2023: (as of this writing, the 2023 Nobel Prize in Physics was awarded to Pierre Agostini, Ferenc Krausz, and Anne L’Huillier for attosecond laser physics – we keep this unchanged, as it’s a cutting-edge field and the recognition seems merit-based).
Finally, looking ahead: Our timeline remains open to acknowledging current under-appreciated areas as their significance comes to light. Some potential “unknown unknowns” (discussed more in Chapter 5) that might warrant future recognition include:
- Quantum computing and information: Pioneers like Peter Shor or David Deutsch might deserve Nobels if and when quantum computers achieve transformative results. In spirit, our timeline already honored Turing and Shannon earlier; similarly, we flag that information science is part of physical science’s evolution, and its heroes deserve credit.
- Emergent phenomena and complexity: If a deep theory unifying complex systems arises (Parisi’s 2021 award was a start), contributors in that domain might be future candidates.
- Interdisciplinary breakthroughs: Perhaps work at the intersection of neuroscience and physics (e.g., a physical theory of consciousness) or biophysics (e.g., physical principles of life) could one day be seen as Nobel-worthy. Our merit framework is open to these once-“fringe” ideas if they prove to unlock fundamental understanding – they would not be excluded just because they don’t fit old categories.
- Global and collaborative science: We foresee that large-scale projects (like the Event Horizon Telescope that imaged a black hole, or international fusion experiments, etc.) might challenge Nobel conventions. Our timeline’s trend has been to increasingly embrace collective awards (as we did explicitly for LIGO 2017, and implicitly for CERN 1984 and others). We predict and advocate that future prizes will come either to consortia or not at all – and a true merit system would find ways to honor the many, not just the few.
In summary, our alternative Nobel timeline from 1901 to 2023 is a richer tapestry than the historical one. It retains the greatness of the original laureates but adds those unjustly omitted. It corrects biases: geographic (more global representation), gender (several women added), theoretical vs experimental (theorists like Noether, Poincaré, Bell recognized alongside or even before experimenters), and structural (posthumous awards and >3 winners allowed to ensure credit where due). It also highlights collaborations as fundamental to modern physics, not as footnotes. This timeline is admittedly idealized – it assumes with hindsight a level of prescience and fairness that real committees might lack. But as an intellectual exercise, it demonstrates concretely how history of science could look if judged purely on the lasting value of contributions.
In the next chapter, we will analyze the patterns of bias and exclusion that the original Nobel history exhibited – many of which our revised timeline had to counteract. This will let us delve deeper into the social and structural factors that caused these merit discrepancies in the first place. We will quantify and discuss gender disparities, geographic concentration, the “rule of three” problem, and other issues, showing how our timeline’s changes directly address them. Such analysis will reinforce why undertaking a merit-only re-evaluation is not just a fanciful exercise, but a revealing mirror held up to science’s culture of recognition.
AI Reasoning
Nobel Prize in Physics: Merit-Only Historical Analysis
Exploring the availability of the original thesis and peer review feedback in user_files to construct the new one.
Searched for True Nobel Physics LaureatesChatGPT
I’m working through the original thesis, "The True Nobel Physics Laureates," which uses a merit-based analysis to list famous scientists and justify their awards. This foundational approach will inform the new thesis, incorporating peer feedback.
Searched for peer review feedback on Nobel physics thesisChatGPT
Now I'm mapping out the need for content on "peer review feedback" and considering alternative searches like "feedback" or "peer" to locate the specific information required.
Searched for peer review on True Nobel Physics LaureatesChatGPT
I'm expanding the historical analysis to highlight overlooked figures and addressing biases to ensure a comprehensive and inclusive thesis on Nobel Prizes merit-based recognition.
Read from Search results for query `The True Nobel Physics Laureates`ChatGPT
Revisiting Nobel's prize equity, addressing overlooked scientists and biases, and suggesting impactful changes for greater recognition.
Navigating Nobel laureates, awards, and institutional biases. Emphasizing key figures and contributions with a focus on overlooked or undervalued scientists.
Searched for Henri Poincaré Nobel Prize nominationChatGPT
I’m compiling citations for overlooked Nobel laureates, biases, cluster prizes, and Nobel rules. Progressing through each, I aim to integrate relevant data and philosophical context.
Searched for scientific recognition and truth quotesChatGPT
I explore philosophical ideas, such as Osler's quote and Planck's reflection, to acknowledge slow recognition and the philosophical relevance of scientific truth and consciousness evolution.
Searched for Michael Faraday Nobel Prize recognitionChatGPT
Adjusting my approach
Searched for Faraday and Poincaré Nobel oversightChatGPT
I'm beginning to see the criteria for Nobel eligibility, which include laureates who hadn't passed away within six months of the announcement.
Read nature.com
ChatGPT
Noticing the snippet mentions Poincaré's 1910 Nobel Prize nominations.
Read pubs.aip.org
ChatGPT
Noticing a snippet about Poincaré's Nobel nominations, considering Geniuses.club for more details.
Read geniuses.club
ChatGPT
I’m honing in on a specific snippet about nominations from geniuses.club, located on lines 25-27. This involves a detailed search within the larger link.
Read more from geniuses.club
ChatGPT
I'm noting Poincaré's broad theoretical contributions versus Nobel's preference for specific discoveries, suggesting a bias towards experimental breakthroughs.
Searched for Lise Meitner Nobel oversight fission
Read physicsworld.com
Read axial.acs.org
ChatGPT
Lise Meitner, even in the domain of chemistry, was unjustly overlooked for the Nobel Prize, likely due to political and gender biases.
Searched for Satyendra Bose Nobel Prize not awardedChatGPT
Interestingly, though Bose's work influenced Nobel awards, he wasn't recognized until much later. Delving into why this was likely involves looking at the timing and societal factors of the era.
Read en.wikipedia.org
ChatGPT
It's interesting to observe that Bose wasn't included among the Nobel Prize recipients for his research contributions. This noteworthiness highlights a significant oversight.
Searched for Chien-Shiung Wu Nobel Prize oversightChatGPT
Focusing on AAUW as an option.
Read aauw.org
ChatGPT
Consulting the AAUW content highlights how Wu's critical contributions in atomic/nuclear physics were overshadowed by her male colleagues' Nobel Prize status.
Read en.wikipedia.org
ChatGPT
Wu, missed Nobel, honored with Wolf Prize in 1978. This gap is crucial for context.
Searched for Claude Shannon information theory and Nobel PrizeChatGPT
It's interesting to see Shannon's achievements, like Dr. Bollobás's honorary degree, highlight his influence in fields without a Nobel Prize category.
Read itsoc.org
Searched for Shannon field with no Nobel PrizeChatGPT
I'm thinking about whether the IEEE Information Theory Society's website requires a handshake to load. If so, I'll explore alternatives like citing the phrase from a search or checking Hacker News.
Read news.ycombinator.com
ChatGPT
Shannon's work earned prestigious honors like the National Medal of Science and the Kyoto Prize, highlighting his pivotal role in information theory, which is crucial for the digital age.
Searched for Alan Turing Nobel recognition in computingChatGPT
Acknowledging computing's unique prize due to the absence of a Nobel category.
Read amturing.acm.org
ChatGPT
Ok, let me see. I'll forge a parallel between the ACM Turing Award and Michael Faraday's pivotal contributions, highlighting their role as foundational figures.
Searched for Michael Faraday as experimental physicistChatGPT
Thinking of depicting their remarkable scientific achievements, possibly labeled "one of the greatest scientists."
Read britannica.com
ChatGPT
I’m considering the omission of Henrietta Leavitt and Vera Rubin, and the gender bias in Nobel recognition. Jocelyn Bell is also a strong example.
Searched for Jocelyn Bell Nobel oversight pulsar
Read theguardian.com
ChatGPT
I’m looking at how the Nobel Prize committee overlooked female scientists for decades, despite their groundbreaking achievements, and exploring team-based recognitions, like the lack of Nobel acknowledgement for the Higgs boson discovery experimenters.
Searched for Nobel Prize three person limit collaborationsChatGPT
Admiring the arguments from Physicsworld and Aeon on Nobel's limits and modern science.
Read physicsworld.com
Read aeon.co
ChatGPT
I'm connecting various Nobel laureates and their collaborators to highlight the importance of scientific recognition and the transient nature of awards compared to the enduring value of truth in science.
Read todayinsci.com
ChatGPT
I’m sorting through the Nobel Prizes in Physics, avoiding political bias, and ensuring fair and diverse recognition of contributors. The goal is a balanced and comprehensive timeline that respects all aspects.
Read geniuses.club
ChatGPT
I’m gathering insights on policy constraints and visual resources. Harnessing graphs and timelines could be the best approach.
Searched for chart of Nobel physics winners by country or genderChatGPT
I'm thinking about their potential chart options, though accessibility without login seems uncertain.
Read statista.com
ChatGPT
I’m piecing together chapters to showcase the Nobel Prize’s biases, consider biases, and possible remedy strategies.
Read more from statista.com
ChatGPT
I’m piecing together the concentration of physics Nobel laureates in Western countries, especially the US, and noting the broader implications of geographic and historical biases in nominations and recognitions.
A young stage manager is overlooked due to her age, highlighting a common bias in the industry.
Read en.wikipedia.org
ChatGPT
The trend in Nobel Prize bias often leaves deserving physicists underfoot. This reflects a need for more equitable recognition.
I’m crafting a clear, concise timeline. Employing sub-lists or bullet points will enhance readability and simplify comprehension.